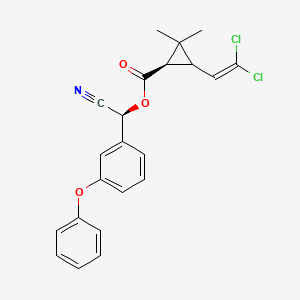
Cypermethrin-beta
Übersicht
Beschreibung
Cypermethrin-beta is a synthetic pyrethroid insecticide widely used in agriculture and pest control. It is a broad-spectrum insecticide that acts by contact and ingestion, targeting a wide range of insect pests. This compound is known for its fast-acting neurotoxic effects on insects, making it an effective tool for pest management .
Vorbereitungsmethoden
Synthetic Routes and Reaction Conditions
Cypermethrin-beta is synthesized through a multi-step process involving the reaction of 3-phenoxybenzyl alcohol with cyanoacetic acid to form the intermediate cyanoacetate ester. This intermediate is then reacted with 2,2-dichlorovinyl-2,2-dimethylcyclopropanecarboxylic acid chloride to produce this compound. The reaction conditions typically involve the use of organic solvents such as dichloromethane and catalysts to facilitate the reactions .
Industrial Production Methods
Industrial production of this compound involves large-scale synthesis using similar reaction pathways as described above. The process is optimized for high yield and purity, with stringent quality control measures to ensure the final product meets regulatory standards. The use of continuous flow reactors and advanced purification techniques such as chromatography are common in industrial settings .
Analyse Chemischer Reaktionen
Types of Reactions
Cypermethrin-beta undergoes various chemical reactions, including:
Oxidation: this compound can be oxidized to form carboxylic acid derivatives.
Hydrolysis: Enzymatic hydrolysis of this compound results in the formation of carboxylic acid metabolites.
Substitution: This compound can undergo nucleophilic substitution reactions, particularly at the cyano group.
Common Reagents and Conditions
Oxidation: Common oxidizing agents include potassium permanganate and hydrogen peroxide.
Hydrolysis: Enzymatic hydrolysis is facilitated by esterases and other hydrolytic enzymes.
Substitution: Nucleophilic reagents such as amines and thiols can be used for substitution reactions.
Major Products Formed
Oxidation: Carboxylic acid derivatives.
Hydrolysis: Carboxylic acid metabolites.
Substitution: Substituted cypermethrin derivatives.
Wissenschaftliche Forschungsanwendungen
Chemistry
Cypermethrin-beta serves as a model compound for studying the reactivity and degradation of pyrethroid insecticides. Its chemical properties allow researchers to investigate the stability and breakdown pathways of similar compounds under various environmental conditions. For instance, studies have shown different degradation patterns of chiral contaminant enantiomers, with beta-cypermethrin being a focal point for understanding these mechanisms .
Biology
In biological research, this compound is examined for its effects on insect physiology and behavior. Recent studies have demonstrated that sublethal concentrations can significantly affect mating behaviors in pests like Helicoverpa armigera, inhibiting sex pheromone production and reducing mating success . Additionally, resistance mechanisms in insects such as Blattella germanica have been explored, revealing insights into metabolic enzyme activities that confer resistance to beta-cypermethrin .
Medicine
The compound is also studied for potential applications in developing new insecticidal formulations. Research has focused on understanding its toxicological effects on non-target organisms and humans. Evaluations of dietary risks associated with beta-cypermethrin exposure have been conducted to ensure safety in agricultural practices .
Industry
In the industrial sector, this compound is employed in formulating pest control products for agriculture, public health, and residential use. Its fast-acting neurotoxic properties make it a preferred choice for controlling pests effectively. The development of nanoemulsions of beta-cypermethrin has been shown to enhance insecticidal efficacy compared to conventional formulations .
Case Study 1: Resistance Mechanisms
A comparative study analyzed resistance rates in Blattella germanica exposed to beta-cypermethrin nanoemulsion versus conventional emulsion. The findings indicated that the nanoemulsion resulted in a slower increase in resistance factors compared to the conventional formulation, suggesting a potential advantage in pest management strategies .
Table 1: Resistance Comparison Between Formulations
Formulation Type | Resistance Factor | Statistical Significance |
---|---|---|
Beta-Cypermethrin Nanoemulsion | Lower rate of increase | p < 0.001 |
Beta-Cypermethrin Emulsion | Higher rate of increase | p < 0.001 |
Case Study 2: Sublethal Effects on Mating
Research on Helicoverpa armigera revealed that sublethal exposure to beta-cypermethrin significantly decreased sex pheromone production, which hindered mating success. This study underscores the ecological implications of pesticide use and highlights the need for integrated pest management strategies that consider non-target effects .
Wirkmechanismus
Cypermethrin-beta exerts its effects by interfering with the central nervous system of insects. It binds to voltage-gated sodium channels, causing prolonged depolarization of nerve cells. This results in muscle spasms, paralysis, and eventually death of the insect. The molecular targets include sodium channels, and the pathways involved are related to the disruption of normal nerve impulse transmission .
Vergleich Mit ähnlichen Verbindungen
Similar Compounds
- Alpha-cypermethrin
- Zeta-cypermethrin
- Deltamethrin
- Permethrin
Comparison
Cypermethrin-beta is unique among pyrethroids due to its specific isomeric composition, which contributes to its high insecticidal activity. Compared to other similar compounds, this compound has a broader spectrum of activity and faster knockdown effects. Its stability and persistence in the environment are also notable, making it a preferred choice for various pest control applications .
Biologische Aktivität
Beta-cypermethrin (BCP) is a synthetic pyrethroid insecticide widely used for pest control in agricultural and domestic settings. Understanding its biological activity is crucial due to its implications for environmental health and toxicology. This article synthesizes findings from various studies, focusing on the biochemical impacts, resistance mechanisms in target organisms, and potential environmental effects.
Beta-cypermethrin exerts its insecticidal effects primarily by disrupting sodium channel function in nerve cells, leading to paralysis and death in insects. This mode of action is similar to other pyrethroids but varies in efficacy based on the target species and environmental conditions.
1.1 Enzymatic Inhibition
Research has demonstrated that BCP inhibits key enzymes involved in neurotransmission, particularly acetylcholinesterase (AChE). In a study on Blattella germanica, significant decreases in AChE activity were observed after exposure to BCP, indicating neurotoxic effects that could impair normal physiological functions .
2.1 In Vivo Studies
In lizards exposed to BCP, various biomarkers were assessed across multiple tissues (e.g., liver, kidney, brain). Significant alterations in enzyme activities such as catalase (CAT) and superoxide dismutase (SOD) were noted, suggesting oxidative stress induced by the compound. For instance, MDA levels increased significantly in liver and kidney tissues after exposure, indicating lipid peroxidation .
Table 1: Enzyme Activity Changes Post-Exposure to Beta-Cypermethrin
Tissue | Enzyme | Activity Change (p-value) |
---|---|---|
Liver | CAT | Increased (p < 0.001) |
Kidney | SOD | Decreased (p = 0.017) |
Brain | AChE | Decreased (p < 0.001) |
Gonad | LDH | Increased (p < 0.001) |
2.2 Human Health Implications
Toxicity studies indicate that BCP can affect human health, particularly concerning the nervous and immune systems. Chronic exposure has been linked to reproductive toxicity and endocrine disruption .
3. Resistance Mechanisms
The development of resistance to beta-cypermethrin has been documented in various insect populations. A comparative study found that resistance factors varied significantly between strains of Blattella germanica treated with BCP nanoemulsion versus conventional emulsion .
Table 2: Resistance Factors in Blattella germanica
Strain | LD50 (Nanoemulsion) | Resistance Factor (Nanoemulsion) | LD50 (Emulsion) | Resistance Factor (Emulsion) |
---|---|---|---|---|
Sensitive | 0.0097 µg/Insect | 1.0000 | 0.0338 µg/Insect | 1.000 |
First Generation | 0.0105 µg/Insect | 1.0825 | 0.0500 µg/Insect | 1.4793 |
Second Generation | 0.0112 µg/Insect | 1.1546 | 0.0625 µg/Insect | 1.8491 |
Third Generation | 0.0124 µg/Insect | 1.2784 | 0.0719 µg/Insect | 2.1272 |
4. Environmental Impact
Beta-cypermethrin poses risks not only to target pests but also to non-target organisms and ecosystems due to its persistence in the environment and potential bioaccumulation.
4.1 Degradation Pathways
Studies have identified microbial degradation pathways for beta-cypermethrin, highlighting the role of specific enzymes capable of breaking down the compound into less harmful metabolites . The identification of these pathways is essential for developing bioremediation strategies.
Eigenschaften
IUPAC Name |
[(S)-cyano-(3-phenoxyphenyl)methyl] (1R)-3-(2,2-dichloroethenyl)-2,2-dimethylcyclopropane-1-carboxylate | |
---|---|---|
Source | PubChem | |
URL | https://pubchem.ncbi.nlm.nih.gov | |
Description | Data deposited in or computed by PubChem | |
InChI |
InChI=1S/C22H19Cl2NO3/c1-22(2)17(12-19(23)24)20(22)21(26)28-18(13-25)14-7-6-10-16(11-14)27-15-8-4-3-5-9-15/h3-12,17-18,20H,1-2H3/t17?,18-,20+/m1/s1 | |
Source | PubChem | |
URL | https://pubchem.ncbi.nlm.nih.gov | |
Description | Data deposited in or computed by PubChem | |
InChI Key |
KAATUXNTWXVJKI-FLXSOZOKSA-N | |
Source | PubChem | |
URL | https://pubchem.ncbi.nlm.nih.gov | |
Description | Data deposited in or computed by PubChem | |
Canonical SMILES |
CC1(C(C1C(=O)OC(C#N)C2=CC(=CC=C2)OC3=CC=CC=C3)C=C(Cl)Cl)C | |
Source | PubChem | |
URL | https://pubchem.ncbi.nlm.nih.gov | |
Description | Data deposited in or computed by PubChem | |
Isomeric SMILES |
CC1([C@@H](C1C=C(Cl)Cl)C(=O)O[C@H](C#N)C2=CC(=CC=C2)OC3=CC=CC=C3)C | |
Source | PubChem | |
URL | https://pubchem.ncbi.nlm.nih.gov | |
Description | Data deposited in or computed by PubChem | |
Molecular Formula |
C22H19Cl2NO3 | |
Source | PubChem | |
URL | https://pubchem.ncbi.nlm.nih.gov | |
Description | Data deposited in or computed by PubChem | |
DSSTOX Substance ID |
DTXSID6052871 | |
Record name | beta-Cypermethrin | |
Source | EPA DSSTox | |
URL | https://comptox.epa.gov/dashboard/DTXSID6052871 | |
Description | DSSTox provides a high quality public chemistry resource for supporting improved predictive toxicology. | |
Molecular Weight |
416.3 g/mol | |
Source | PubChem | |
URL | https://pubchem.ncbi.nlm.nih.gov | |
Description | Data deposited in or computed by PubChem | |
CAS No. |
1224510-29-5 | |
Record name | beta-Cypermethrin [ISO] | |
Source | ChemIDplus | |
URL | https://pubchem.ncbi.nlm.nih.gov/substance/?source=chemidplus&sourceid=1224510295 | |
Description | ChemIDplus is a free, web search system that provides access to the structure and nomenclature authority files used for the identification of chemical substances cited in National Library of Medicine (NLM) databases, including the TOXNET system. | |
Record name | beta-Cypermethrin | |
Source | EPA DSSTox | |
URL | https://comptox.epa.gov/dashboard/DTXSID6052871 | |
Description | DSSTox provides a high quality public chemistry resource for supporting improved predictive toxicology. | |
Synthesis routes and methods I
Procedure details
Synthesis routes and methods II
Procedure details
Synthesis routes and methods III
Procedure details
Synthesis routes and methods IV
Procedure details
Retrosynthesis Analysis
AI-Powered Synthesis Planning: Our tool employs the Template_relevance Pistachio, Template_relevance Bkms_metabolic, Template_relevance Pistachio_ringbreaker, Template_relevance Reaxys, Template_relevance Reaxys_biocatalysis model, leveraging a vast database of chemical reactions to predict feasible synthetic routes.
One-Step Synthesis Focus: Specifically designed for one-step synthesis, it provides concise and direct routes for your target compounds, streamlining the synthesis process.
Accurate Predictions: Utilizing the extensive PISTACHIO, BKMS_METABOLIC, PISTACHIO_RINGBREAKER, REAXYS, REAXYS_BIOCATALYSIS database, our tool offers high-accuracy predictions, reflecting the latest in chemical research and data.
Strategy Settings
Precursor scoring | Relevance Heuristic |
---|---|
Min. plausibility | 0.01 |
Model | Template_relevance |
Template Set | Pistachio/Bkms_metabolic/Pistachio_ringbreaker/Reaxys/Reaxys_biocatalysis |
Top-N result to add to graph | 6 |
Feasible Synthetic Routes
Haftungsausschluss und Informationen zu In-Vitro-Forschungsprodukten
Bitte beachten Sie, dass alle Artikel und Produktinformationen, die auf BenchChem präsentiert werden, ausschließlich zu Informationszwecken bestimmt sind. Die auf BenchChem zum Kauf angebotenen Produkte sind speziell für In-vitro-Studien konzipiert, die außerhalb lebender Organismen durchgeführt werden. In-vitro-Studien, abgeleitet von dem lateinischen Begriff "in Glas", beinhalten Experimente, die in kontrollierten Laborumgebungen unter Verwendung von Zellen oder Geweben durchgeführt werden. Es ist wichtig zu beachten, dass diese Produkte nicht als Arzneimittel oder Medikamente eingestuft sind und keine Zulassung der FDA für die Vorbeugung, Behandlung oder Heilung von medizinischen Zuständen, Beschwerden oder Krankheiten erhalten haben. Wir müssen betonen, dass jede Form der körperlichen Einführung dieser Produkte in Menschen oder Tiere gesetzlich strikt untersagt ist. Es ist unerlässlich, sich an diese Richtlinien zu halten, um die Einhaltung rechtlicher und ethischer Standards in Forschung und Experiment zu gewährleisten.