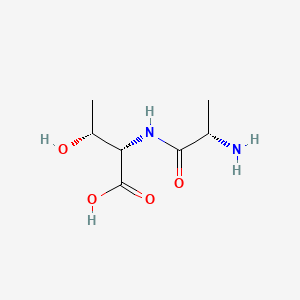
L-alanyl-L-threonine
Übersicht
Beschreibung
L-alanyl-L-threonine, also known as Ala-Thr, is a dipeptide formed from L-alanyl and L-threonine residues . It plays a role as a metabolite and is a tautomer of an Ala-Thr zwitterion .
Synthesis Analysis
The biosynthesis pathway of L-threonine in Escherichia coli has been studied extensively . The industrial production of L-threonine is based on direct fermentation with microorganisms such as Escherichia coli . This process has the characteristics of low cost and high productivity .Molecular Structure Analysis
The molecular formula of L-alanyl-L-threonine is C7H14N2O4 . Its molecular weight is 190.20 g/mol . The crystal structure of the compound contains an intramolecular hydrogen bond .Chemical Reactions Analysis
The effects of L-theanine parallel those of GABA neurotransmission, which regulates a delicate balance between activation and inhibition . These inhibitory signals are vital for mood, memory, attention, and relaxation in our cognitive framework .Physical And Chemical Properties Analysis
L-alanyl-L-threonine is a dipeptide formed from L-alanyl and L-threonine residues . It is a tautomer of an Ala-Thr zwitterion .Wissenschaftliche Forschungsanwendungen
Crystallography Studies
L-alanyl-L-threonine has been used in crystallography studies . The crystal and molecular structure of this compound provides valuable insights into its properties and potential applications.
Microbial Physiology and Metabolism
In microbial physiology and metabolism, L-alanyl-L-threonine plays a significant role. For instance, it has been studied for its uptake in Escherichia coli K-12 . The YifK gene product acts as a permease specific to L-threonine and, to a lesser extent, L-serine .
Cryoprotectant
L-alanyl-L-threonine has been studied as a biomimetic cryoprotectant . It has potential applications in the preservation of biological samples at low temperatures.
Metabolic Engineering
L-alanyl-L-threonine has been used in metabolic engineering of Escherichia coli for efficient production of L-alanyl-L-glutamine . This involves over-expressing L-amino acid α-ligase (BacD) from Bacillus subtilis, and inactivating certain peptidases and the dipeptide transport system Dpp .
Amino Acid Carrier
L-alanyl-L-threonine has been identified as a novel amino acid carrier in E. coli K-12 cells . It utilizes a proton motive force to energize substrate uptake .
Threonine/Serine Uptake Subsystem
L-alanyl-L-threonine is part of the threonine/serine uptake subsystem in E. coli cells . This subsystem is key to understanding how cellular metabolic and regulatory networks interact with the external environment and respond to changes in medium composition .
Wirkmechanismus
Target of Action
L-alanyl-L-threonine, also known as H-ALA-THR-OH, is a dipeptide consisting of the amino acids alanine and threonine It’s worth noting that dipeptides like l-alanyl-l-glutamine have been shown to target various cellular processes, including muscle recovery and inflammation regulation .
Mode of Action
For instance, L-alanyl-L-glutamine has been shown to reduce bacterial translocation, thus reducing the risk of infections and infection-related problems .
Biochemical Pathways
For instance, L-alanyl-L-glutamine has been shown to play a key role in several essential metabolic processes and is an important modulator of the heat shock protein (HSP) response .
Pharmacokinetics
It’s worth noting that l-alanyl-l-glutamine, a similar dipeptide, has high water solubility, good thermal stability, and high bioavailability, which are desirable properties for a compound used in dietary supplementation, parenteral nutrition, and cell culture .
Result of Action
Similar dipeptides like l-alanyl-l-glutamine have been shown to have protective effects on the gastrointestinal tract, reducing the risk of infections and infection-related problems such as diarrhea, dehydration, malabsorption, and electrolyte imbalance .
Action Environment
It’s worth noting that similar dipeptides like l-alanyl-l-glutamine have been shown to be stable in aqueous solution, unlike l-glutamine which spontaneously degrades to form ammonia and pyrrolidine carboxylic acid .
Safety and Hazards
L-alanyl-L-threonine should be handled with care. Avoid breathing mist, gas or vapours. Avoid contacting with skin and eye. Use personal protective equipment. Wear chemical impermeable gloves. Ensure adequate ventilation. Remove all sources of ignition. Evacuate personnel to safe areas. Keep people away from and upwind of spill/leak .
Eigenschaften
IUPAC Name |
(2S,3R)-2-[[(2S)-2-aminopropanoyl]amino]-3-hydroxybutanoic acid | |
---|---|---|
Source | PubChem | |
URL | https://pubchem.ncbi.nlm.nih.gov | |
Description | Data deposited in or computed by PubChem | |
InChI |
InChI=1S/C7H14N2O4/c1-3(8)6(11)9-5(4(2)10)7(12)13/h3-5,10H,8H2,1-2H3,(H,9,11)(H,12,13)/t3-,4+,5-/m0/s1 | |
Source | PubChem | |
URL | https://pubchem.ncbi.nlm.nih.gov | |
Description | Data deposited in or computed by PubChem | |
InChI Key |
BUQICHWNXBIBOG-LMVFSUKVSA-N | |
Source | PubChem | |
URL | https://pubchem.ncbi.nlm.nih.gov | |
Description | Data deposited in or computed by PubChem | |
Canonical SMILES |
CC(C(C(=O)O)NC(=O)C(C)N)O | |
Source | PubChem | |
URL | https://pubchem.ncbi.nlm.nih.gov | |
Description | Data deposited in or computed by PubChem | |
Isomeric SMILES |
C[C@H]([C@@H](C(=O)O)NC(=O)[C@H](C)N)O | |
Source | PubChem | |
URL | https://pubchem.ncbi.nlm.nih.gov | |
Description | Data deposited in or computed by PubChem | |
Molecular Formula |
C7H14N2O4 | |
Source | PubChem | |
URL | https://pubchem.ncbi.nlm.nih.gov | |
Description | Data deposited in or computed by PubChem | |
DSSTOX Substance ID |
DTXSID30178781 | |
Record name | Dipeptide-14 | |
Source | EPA DSSTox | |
URL | https://comptox.epa.gov/dashboard/DTXSID30178781 | |
Description | DSSTox provides a high quality public chemistry resource for supporting improved predictive toxicology. | |
Molecular Weight |
190.20 g/mol | |
Source | PubChem | |
URL | https://pubchem.ncbi.nlm.nih.gov | |
Description | Data deposited in or computed by PubChem | |
Physical Description |
Solid | |
Record name | Alanylthreonine | |
Source | Human Metabolome Database (HMDB) | |
URL | http://www.hmdb.ca/metabolites/HMDB0028697 | |
Description | The Human Metabolome Database (HMDB) is a freely available electronic database containing detailed information about small molecule metabolites found in the human body. | |
Explanation | HMDB is offered to the public as a freely available resource. Use and re-distribution of the data, in whole or in part, for commercial purposes requires explicit permission of the authors and explicit acknowledgment of the source material (HMDB) and the original publication (see the HMDB citing page). We ask that users who download significant portions of the database cite the HMDB paper in any resulting publications. | |
Product Name |
L-alanyl-L-threonine | |
CAS RN |
24032-50-6 | |
Record name | Dipeptide-14 | |
Source | ChemIDplus | |
URL | https://pubchem.ncbi.nlm.nih.gov/substance/?source=chemidplus&sourceid=0024032506 | |
Description | ChemIDplus is a free, web search system that provides access to the structure and nomenclature authority files used for the identification of chemical substances cited in National Library of Medicine (NLM) databases, including the TOXNET system. | |
Record name | Dipeptide-14 | |
Source | EPA DSSTox | |
URL | https://comptox.epa.gov/dashboard/DTXSID30178781 | |
Description | DSSTox provides a high quality public chemistry resource for supporting improved predictive toxicology. | |
Record name | ALANYLTHREONINE | |
Source | FDA Global Substance Registration System (GSRS) | |
URL | https://gsrs.ncats.nih.gov/ginas/app/beta/substances/KJQ8278D0V | |
Description | The FDA Global Substance Registration System (GSRS) enables the efficient and accurate exchange of information on what substances are in regulated products. Instead of relying on names, which vary across regulatory domains, countries, and regions, the GSRS knowledge base makes it possible for substances to be defined by standardized, scientific descriptions. | |
Explanation | Unless otherwise noted, the contents of the FDA website (www.fda.gov), both text and graphics, are not copyrighted. They are in the public domain and may be republished, reprinted and otherwise used freely by anyone without the need to obtain permission from FDA. Credit to the U.S. Food and Drug Administration as the source is appreciated but not required. | |
Record name | Alanylthreonine | |
Source | Human Metabolome Database (HMDB) | |
URL | http://www.hmdb.ca/metabolites/HMDB0028697 | |
Description | The Human Metabolome Database (HMDB) is a freely available electronic database containing detailed information about small molecule metabolites found in the human body. | |
Explanation | HMDB is offered to the public as a freely available resource. Use and re-distribution of the data, in whole or in part, for commercial purposes requires explicit permission of the authors and explicit acknowledgment of the source material (HMDB) and the original publication (see the HMDB citing page). We ask that users who download significant portions of the database cite the HMDB paper in any resulting publications. | |
Retrosynthesis Analysis
AI-Powered Synthesis Planning: Our tool employs the Template_relevance Pistachio, Template_relevance Bkms_metabolic, Template_relevance Pistachio_ringbreaker, Template_relevance Reaxys, Template_relevance Reaxys_biocatalysis model, leveraging a vast database of chemical reactions to predict feasible synthetic routes.
One-Step Synthesis Focus: Specifically designed for one-step synthesis, it provides concise and direct routes for your target compounds, streamlining the synthesis process.
Accurate Predictions: Utilizing the extensive PISTACHIO, BKMS_METABOLIC, PISTACHIO_RINGBREAKER, REAXYS, REAXYS_BIOCATALYSIS database, our tool offers high-accuracy predictions, reflecting the latest in chemical research and data.
Strategy Settings
Precursor scoring | Relevance Heuristic |
---|---|
Min. plausibility | 0.01 |
Model | Template_relevance |
Template Set | Pistachio/Bkms_metabolic/Pistachio_ringbreaker/Reaxys/Reaxys_biocatalysis |
Top-N result to add to graph | 6 |
Feasible Synthetic Routes
Q & A
Q1: Why is L-alanyl-L-threonine important in microbiological research?
A1: L-alanyl-L-threonine serves as a valuable tool in understanding amino acid transport mechanisms in bacteria. For instance, researchers have utilized L-alanyl-L-threonine auxotrophy to investigate L-threonine uptake in Escherichia coli. [] They discovered that specific mutants, unable to grow on L-threonine alone, could grow when provided with L-alanyl-L-threonine. This finding suggests that L-alanyl-L-threonine utilizes a distinct transport system compared to L-threonine, highlighting the complexity of nutrient uptake in bacteria.
Q2: How does L-alanyl-L-threonine contribute to our understanding of bacterial metabolism?
A2: L-alanyl-L-threonine plays a role in characterizing novel bacterial species. For example, Murimonas intestini, a bacterium isolated from the mouse gut, was found to utilize L-alanyl-L-threonine as an energy source. [] This metabolic capability, alongside other phenotypic and genotypic characteristics, helped distinguish M. intestini as a novel species within the Lachnospiraceae family.
Q3: What is the structural significance of the L-alanyl-L-threonine molecule?
A3: The crystal structure of L-alanyl-L-threonine reveals a unique intramolecular interaction. [] The side-chain hydroxyl group of the L-threonine residue forms a hydrogen bond with the main-chain carboxylate group. This specific interaction, observed for the first time in a C-terminal L-threonine residue, provides insights into the conformational preferences and stability of peptides containing L-threonine at their C-terminus.
Q4: Can you elaborate on the research surrounding L-threonine production in E. coli and the role of L-alanyl-L-threonine?
A4: Scientists have successfully engineered E. coli strains capable of hyperproducing L-threonine, a valuable amino acid for various applications. [] Interestingly, these strains exhibited impaired L-threonine uptake. Researchers utilized L-alanyl-L-threonine and glycyl-L-threonine auxotrophic mutants to demonstrate that the engineered strains could grow in the presence of low dipeptide concentrations but required significantly higher L-threonine concentrations. This observation suggests that the hyperproduction mechanism involves reduced L-threonine uptake, limiting feedback inhibition and ultimately increasing L-threonine production. []
Q5: How does understanding the transport of dipeptides like L-alanyl-L-threonine benefit biotechnological applications?
A5: Unraveling the mechanisms behind dipeptide transport in bacteria like E. coli has significant implications for biotechnological processes. [] By manipulating these transport systems, researchers can potentially enhance the production of valuable compounds like L-threonine. Understanding the distinct uptake pathways of dipeptides compared to their constituent amino acids offers valuable tools for optimizing microbial fermentation and bioproduction strategies.
Haftungsausschluss und Informationen zu In-Vitro-Forschungsprodukten
Bitte beachten Sie, dass alle Artikel und Produktinformationen, die auf BenchChem präsentiert werden, ausschließlich zu Informationszwecken bestimmt sind. Die auf BenchChem zum Kauf angebotenen Produkte sind speziell für In-vitro-Studien konzipiert, die außerhalb lebender Organismen durchgeführt werden. In-vitro-Studien, abgeleitet von dem lateinischen Begriff "in Glas", beinhalten Experimente, die in kontrollierten Laborumgebungen unter Verwendung von Zellen oder Geweben durchgeführt werden. Es ist wichtig zu beachten, dass diese Produkte nicht als Arzneimittel oder Medikamente eingestuft sind und keine Zulassung der FDA für die Vorbeugung, Behandlung oder Heilung von medizinischen Zuständen, Beschwerden oder Krankheiten erhalten haben. Wir müssen betonen, dass jede Form der körperlichen Einführung dieser Produkte in Menschen oder Tiere gesetzlich strikt untersagt ist. Es ist unerlässlich, sich an diese Richtlinien zu halten, um die Einhaltung rechtlicher und ethischer Standards in Forschung und Experiment zu gewährleisten.