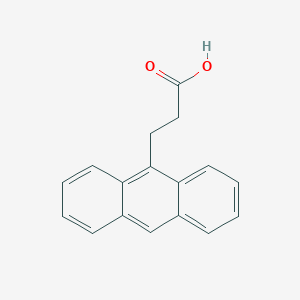
9-Anthracenpropionsäure
Übersicht
Beschreibung
9-Anthracenepropionic acid: is an organic compound that belongs to the class of anthracene derivatives. Anthracene is a polycyclic aromatic hydrocarbon consisting of three fused benzene rings. The propionic acid moiety is attached to the ninth position of the anthracene ring system. This compound is of interest due to its unique photophysical and photochemical properties, making it valuable in various scientific and industrial applications.
Wissenschaftliche Forschungsanwendungen
Chemistry: 9-Anthracenepropionic acid is used as a precursor in the synthesis of various anthracene-based compounds. Its unique photophysical properties make it valuable in the study of photochemical reactions and the development of organic light-emitting diodes (OLEDs).
Biology: In biological research, 9-Anthracenepropionic acid is used as a fluorescent probe due to its strong fluorescence. It is employed in the study of cellular processes and the detection of biomolecules.
Medicine: The compound has potential applications in medicine, particularly in the development of photodynamic therapy agents. Its ability to generate reactive oxygen species upon irradiation makes it useful in targeting cancer cells.
Industry: In the industrial sector, 9-Anthracenepropionic acid is used in the production of dyes, pigments, and other organic materials. Its stability and photophysical properties make it suitable for various applications.
Safety and Hazards
Zukünftige Richtungen
The photogeneration of stable radicals is important but still challenging in the field of optical switching, displays, and other devices . 9-Anthracenepropionic acid and a mononuclear complex constructed from this ligand were discovered to show radical-induced photochromism and photomagnetism after Xe lamp light irradiation . This study finds a simple radical-actuated photochromic molecule for constructing a novel system of photochromic materials .
Wirkmechanismus
Target of Action
It’s known that anthracene derivatives have been commonly utilized in the construction of coordination complexes .
Mode of Action
Anthracene derivatives are known to undergo intermolecular [4+4] cycloaddition reactions after uv light irradiation . This reaction can induce mechanical bending, magnetic, and photoluminescent variations .
Biochemical Pathways
Anthracene derivatives are known to participate in photochemical reactions . For instance, the photodimerization of the 9-Anthracenepropionic acid ligand has been studied as it is prone to intermolecular [4+4] cycloaddition reactions after UV light irradiation .
Result of Action
It’s known that anthracene derivatives can show radical-induced photochromism and photomagnetism after xe lamp light irradiation . This suggests that 9-Anthracenepropionic acid may have similar properties.
Action Environment
The action of 9-Anthracenepropionic acid can be influenced by environmental factors such as light. For instance, anthracene derivatives are known to undergo photochemical reactions upon light irradiation . Therefore, the presence and intensity of light can significantly influence the action, efficacy, and stability of 9-Anthracenepropionic acid.
Biochemische Analyse
Biochemical Properties
9-Anthracenepropionic acid plays a significant role in biochemical reactions, particularly in the formation of coordination complexes. It interacts with various enzymes, proteins, and other biomolecules. For instance, it has been observed to participate in radical-induced photochromism and photomagnetism when exposed to light irradiation . The compound’s large π-conjugated system allows it to engage in electron transfer processes, making it a valuable component in the design of photochromic materials . Additionally, 9-Anthracenepropionic acid can undergo intermolecular [4+4] cycloaddition reactions, which are crucial for its photodimerization properties .
Cellular Effects
The effects of 9-Anthracenepropionic acid on various types of cells and cellular processes are profound. It influences cell function by modulating cell signaling pathways, gene expression, and cellular metabolism. The compound’s ability to participate in electron transfer processes can impact cellular redox states, thereby affecting various metabolic pathways . Moreover, its interaction with cellular proteins can lead to changes in gene expression, influencing cell proliferation and differentiation.
Molecular Mechanism
At the molecular level, 9-Anthracenepropionic acid exerts its effects through several mechanisms. It can bind to specific biomolecules, leading to enzyme inhibition or activation. The compound’s ability to undergo photochromic changes upon light irradiation suggests that it can act as a molecular switch, altering its conformation and activity in response to external stimuli . This property is particularly useful in the development of dynamic optical materials and molecular devices.
Temporal Effects in Laboratory Settings
In laboratory settings, the effects of 9-Anthracenepropionic acid can change over time. The compound’s stability and degradation are influenced by factors such as light exposure and temperature. Studies have shown that 9-Anthracenepropionic acid can form stable radicals upon light irradiation, which can revert to their original state through heat treatment or further light exposure . These reversible changes are essential for its application in dynamic optical crosslinking and energy storage systems.
Dosage Effects in Animal Models
The effects of 9-Anthracenepropionic acid vary with different dosages in animal models. At lower doses, the compound may exhibit minimal toxicity and can be used to study its biochemical properties without adverse effects. At higher doses, 9-Anthracenepropionic acid may induce toxic effects, including oxidative stress and cellular damage . It is crucial to determine the appropriate dosage to balance its beneficial properties with potential adverse effects.
Metabolic Pathways
9-Anthracenepropionic acid is involved in various metabolic pathways, interacting with enzymes and cofactors. Its role in electron transfer processes suggests that it can influence metabolic flux and alter metabolite levels . The compound’s ability to undergo photodimerization and other chemical reactions further highlights its involvement in complex biochemical pathways.
Transport and Distribution
Within cells and tissues, 9-Anthracenepropionic acid is transported and distributed through interactions with specific transporters and binding proteins. These interactions can affect its localization and accumulation in different cellular compartments . The compound’s solubility in organic solvents and limited solubility in water also influence its distribution within biological systems .
Subcellular Localization
The subcellular localization of 9-Anthracenepropionic acid is determined by its chemical properties and interactions with cellular components. It may be directed to specific compartments or organelles through targeting signals or post-translational modifications
Vorbereitungsmethoden
Synthetic Routes and Reaction Conditions: The synthesis of 9-Anthracenepropionic acid typically involves the following steps:
Friedel-Crafts Alkylation: Anthracene undergoes Friedel-Crafts alkylation with propionic acid or its derivatives in the presence of a Lewis acid catalyst such as aluminum chloride.
Oxidation: The resulting intermediate is then oxidized to form 9-Anthracenepropionic acid. Common oxidizing agents include potassium permanganate or chromium trioxide.
Industrial Production Methods: Industrial production methods for 9-Anthracenepropionic acid are similar to laboratory synthesis but are scaled up to accommodate larger quantities. The use of continuous flow reactors and optimized reaction conditions ensures higher yields and purity.
Analyse Chemischer Reaktionen
Types of Reactions:
Oxidation: 9-Anthracenepropionic acid can undergo oxidation reactions to form various oxidized derivatives. Common oxidizing agents include potassium permanganate and chromium trioxide.
Reduction: Reduction reactions can convert 9-Anthracenepropionic acid to its corresponding alcohol or other reduced forms. Sodium borohydride and lithium aluminum hydride are typical reducing agents.
Substitution: The compound can undergo electrophilic substitution reactions, where the propionic acid moiety can be replaced with other functional groups. Common reagents include halogens and nitrating agents.
Common Reagents and Conditions:
Oxidation: Potassium permanganate, chromium trioxide.
Reduction: Sodium borohydride, lithium aluminum hydride.
Substitution: Halogens, nitrating agents.
Major Products Formed:
Oxidation: Oxidized derivatives such as anthraquinone derivatives.
Reduction: Reduced forms such as alcohols.
Substitution: Various substituted anthracene derivatives.
Vergleich Mit ähnlichen Verbindungen
9-Anthracenemethanol: Similar in structure but with a hydroxyl group instead of a propionic acid moiety.
9-Anthracenecarboxylic acid: Contains a carboxylic acid group at the ninth position instead of a propionic acid moiety.
9,10-Diphenylanthracene: Substituted with phenyl groups at the ninth and tenth positions.
Uniqueness: 9-Anthracenepropionic acid is unique due to its combination of the anthracene core and the propionic acid moiety. This combination imparts distinct photophysical properties, making it valuable in applications requiring strong fluorescence and photochemical reactivity. Its ability to generate reactive oxygen species upon irradiation sets it apart from other similar compounds, making it particularly useful in photodynamic therapy and other light-induced applications.
Eigenschaften
IUPAC Name |
3-anthracen-9-ylpropanoic acid | |
---|---|---|
Source | PubChem | |
URL | https://pubchem.ncbi.nlm.nih.gov | |
Description | Data deposited in or computed by PubChem | |
InChI |
InChI=1S/C17H14O2/c18-17(19)10-9-16-14-7-3-1-5-12(14)11-13-6-2-4-8-15(13)16/h1-8,11H,9-10H2,(H,18,19) | |
Source | PubChem | |
URL | https://pubchem.ncbi.nlm.nih.gov | |
Description | Data deposited in or computed by PubChem | |
InChI Key |
BDGMYCZUEIGHJH-UHFFFAOYSA-N | |
Source | PubChem | |
URL | https://pubchem.ncbi.nlm.nih.gov | |
Description | Data deposited in or computed by PubChem | |
Canonical SMILES |
C1=CC=C2C(=C1)C=C3C=CC=CC3=C2CCC(=O)O | |
Source | PubChem | |
URL | https://pubchem.ncbi.nlm.nih.gov | |
Description | Data deposited in or computed by PubChem | |
Molecular Formula |
C17H14O2 | |
Source | PubChem | |
URL | https://pubchem.ncbi.nlm.nih.gov | |
Description | Data deposited in or computed by PubChem | |
DSSTOX Substance ID |
DTXSID70194006 | |
Record name | 9-Anthracenepropionic acid | |
Source | EPA DSSTox | |
URL | https://comptox.epa.gov/dashboard/DTXSID70194006 | |
Description | DSSTox provides a high quality public chemistry resource for supporting improved predictive toxicology. | |
Molecular Weight |
250.29 g/mol | |
Source | PubChem | |
URL | https://pubchem.ncbi.nlm.nih.gov | |
Description | Data deposited in or computed by PubChem | |
CAS No. |
41034-83-7 | |
Record name | 9-Anthracenepropionic acid | |
Source | ChemIDplus | |
URL | https://pubchem.ncbi.nlm.nih.gov/substance/?source=chemidplus&sourceid=0041034837 | |
Description | ChemIDplus is a free, web search system that provides access to the structure and nomenclature authority files used for the identification of chemical substances cited in National Library of Medicine (NLM) databases, including the TOXNET system. | |
Record name | 9-Anthracenepropionic acid | |
Source | EPA DSSTox | |
URL | https://comptox.epa.gov/dashboard/DTXSID70194006 | |
Description | DSSTox provides a high quality public chemistry resource for supporting improved predictive toxicology. | |
Retrosynthesis Analysis
AI-Powered Synthesis Planning: Our tool employs the Template_relevance Pistachio, Template_relevance Bkms_metabolic, Template_relevance Pistachio_ringbreaker, Template_relevance Reaxys, Template_relevance Reaxys_biocatalysis model, leveraging a vast database of chemical reactions to predict feasible synthetic routes.
One-Step Synthesis Focus: Specifically designed for one-step synthesis, it provides concise and direct routes for your target compounds, streamlining the synthesis process.
Accurate Predictions: Utilizing the extensive PISTACHIO, BKMS_METABOLIC, PISTACHIO_RINGBREAKER, REAXYS, REAXYS_BIOCATALYSIS database, our tool offers high-accuracy predictions, reflecting the latest in chemical research and data.
Strategy Settings
Precursor scoring | Relevance Heuristic |
---|---|
Min. plausibility | 0.01 |
Model | Template_relevance |
Template Set | Pistachio/Bkms_metabolic/Pistachio_ringbreaker/Reaxys/Reaxys_biocatalysis |
Top-N result to add to graph | 6 |
Feasible Synthetic Routes
Q1: Why is 9-Anthracenepropionic acid being used to modify single-walled carbon nanotubes (SWCNTs)?
A1: The carboxylic acid group present in 9-Anthracenepropionic acid enables it to interact with SWCNTs. [] This interaction allows the modified SWCNTs to disperse stably in water, a crucial property for creating conductive thin films via techniques like spray-coating. [] The anthracene moiety likely contributes to the dispersion through its hydrophobic interactions with the SWCNTs.
Q2: How does the presence of water affect the sorption of 9-Anthracenepropionic acid in soil?
A2: Research suggests that water plays a significant role in the "non-labile" uptake of 9-Anthracenepropionic acid in both clean and oil-contaminated soils. [] This non-labile uptake refers to the portion of the compound that doesn't readily desorb and may indicate stronger interactions with the soil matrix. Interestingly, water-repellent soils did not exhibit this non-labile uptake, further highlighting the importance of water in this process. []
Q3: Can 9-Anthracenepropionic acid be used to model the behavior of other contaminants in the environment?
A3: Yes, 9-Anthracenepropionic acid has been employed as a model compound to understand the behavior of polycyclic aromatic hydrocarbons (PAHs) in the gastrointestinal tract. [] Its release from a contaminated soil matrix within a simulated digestive system was studied to understand how such contaminants might become bioavailable after soil ingestion. [] The research suggests that while initial release from the soil matrix might be facilitated in the presence of bile salts and organic matter, subsequent complexation can reduce the freely dissolved fraction. []
Haftungsausschluss und Informationen zu In-Vitro-Forschungsprodukten
Bitte beachten Sie, dass alle Artikel und Produktinformationen, die auf BenchChem präsentiert werden, ausschließlich zu Informationszwecken bestimmt sind. Die auf BenchChem zum Kauf angebotenen Produkte sind speziell für In-vitro-Studien konzipiert, die außerhalb lebender Organismen durchgeführt werden. In-vitro-Studien, abgeleitet von dem lateinischen Begriff "in Glas", beinhalten Experimente, die in kontrollierten Laborumgebungen unter Verwendung von Zellen oder Geweben durchgeführt werden. Es ist wichtig zu beachten, dass diese Produkte nicht als Arzneimittel oder Medikamente eingestuft sind und keine Zulassung der FDA für die Vorbeugung, Behandlung oder Heilung von medizinischen Zuständen, Beschwerden oder Krankheiten erhalten haben. Wir müssen betonen, dass jede Form der körperlichen Einführung dieser Produkte in Menschen oder Tiere gesetzlich strikt untersagt ist. Es ist unerlässlich, sich an diese Richtlinien zu halten, um die Einhaltung rechtlicher und ethischer Standards in Forschung und Experiment zu gewährleisten.