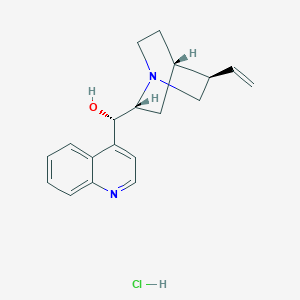
Cinchonine hydrochloride
- Klicken Sie auf QUICK INQUIRY, um ein Angebot von unserem Expertenteam zu erhalten.
- Mit qualitativ hochwertigen Produkten zu einem WETTBEWERBSFÄHIGEN Preis können Sie sich mehr auf Ihre Forschung konzentrieren.
Übersicht
Beschreibung
Cinchonine hydrochloride is a quinoline alkaloid derived from the bark of the Cinchona tree. It is one of the major alkaloids found in Cinchona species, alongside quinine, quinidine, and cinchonidine . This compound is known for its diverse biological activities and has been used historically for its antimalarial properties .
Biochemische Analyse
Biochemical Properties
Cinchonine hydrochloride plays a significant role in biochemical reactions, particularly in its interactions with enzymes, proteins, and other biomolecules. It is known to interact with the glucagon-like peptide-1 (GLP-1) receptor, acting as an agonist . This interaction is crucial for its potential therapeutic effects in treating conditions such as obesity, type 2 diabetes, and non-alcoholic fatty liver disease. Additionally, this compound has been shown to inhibit human platelet aggregation by blocking calcium influx .
Cellular Effects
This compound influences various cellular processes and functions. It has been observed to affect cell signaling pathways, gene expression, and cellular metabolism. For instance, it can ameliorate hyperlipidemia and hyperglycemia induced by a high-fat diet, which are early symptoms of metabolic syndrome . This compound also exhibits anti-inflammatory properties by down-regulating WNT and galanin-mediated adipogenesis signaling pathways .
Molecular Mechanism
The molecular mechanism of this compound involves its binding interactions with biomolecules and its ability to modulate enzyme activity. As a GLP-1 receptor agonist, it binds to the receptor and activates downstream signaling pathways that regulate glucose metabolism and insulin secretion . Additionally, this compound inhibits calcium influx in human platelets, thereby preventing platelet aggregation .
Temporal Effects in Laboratory Settings
In laboratory settings, the effects of this compound can change over time. The compound’s stability and degradation are important factors to consider. This compound is known to be unstable and susceptible to deliquescence, which can affect its long-term efficacy . Studies have shown that its stability can be enhanced by forming cocrystal salts or other stable derivatives .
Dosage Effects in Animal Models
The effects of this compound vary with different dosages in animal models. At therapeutic doses, it has been shown to effectively lower blood glucose levels and improve metabolic parameters . At higher doses, it may exhibit toxic or adverse effects, such as gastrointestinal disturbances and potential hepatotoxicity . It is crucial to determine the optimal dosage to maximize therapeutic benefits while minimizing adverse effects.
Metabolic Pathways
This compound is involved in various metabolic pathways. It undergoes biotransformation in the liver, where it is metabolized by cytochrome P450 enzymes, particularly CYP3A4 . This metabolic pathway leads to the formation of glucuronides, which are then excreted from the body. The compound’s interaction with metabolic enzymes can influence its pharmacokinetics and overall efficacy.
Transport and Distribution
The transport and distribution of this compound within cells and tissues are mediated by specific transporters and binding proteins. It is known to interact with membrane transporters that facilitate its uptake into cells . Once inside the cells, this compound can accumulate in specific tissues, influencing its localization and therapeutic effects.
Subcellular Localization
This compound’s subcellular localization plays a crucial role in its activity and function. It is primarily localized in the cytoplasm and nucleus, where it can interact with various cellular components . The compound’s localization is influenced by targeting signals and post-translational modifications that direct it to specific compartments or organelles within the cell.
Vorbereitungsmethoden
Synthetic Routes and Reaction Conditions
Cinchonine hydrochloride can be synthesized through the extraction of cinchonine from Cinchona bark, followed by its conversion to the hydrochloride salt. The extraction process typically involves the use of organic solvents to isolate cinchonine, which is then reacted with hydrochloric acid to form this compound .
Industrial Production Methods
Industrial production of this compound involves large-scale extraction from Cinchona bark, followed by purification and crystallization processes. The extracted cinchonine is treated with hydrochloric acid to produce this compound, which is then purified through recrystallization .
Analyse Chemischer Reaktionen
Types of Reactions
Cinchonine hydrochloride undergoes various chemical reactions, including:
Oxidation: Cinchonine can be oxidized to form various derivatives.
Reduction: Reduction reactions can modify the quinoline ring structure.
Substitution: Substitution reactions can occur at different positions on the quinoline ring.
Common Reagents and Conditions
Common reagents used in these reactions include oxidizing agents like potassium permanganate, reducing agents like sodium borohydride, and various nucleophiles for substitution reactions .
Major Products Formed
The major products formed from these reactions include oxidized derivatives, reduced forms of cinchonine, and substituted quinoline compounds .
Wissenschaftliche Forschungsanwendungen
Cinchonine hydrochloride has a wide range of scientific research applications:
Chemistry: It is used as a chiral catalyst in asymmetric synthesis and as a chiral stationary phase in chromatography.
Biology: This compound exhibits antimalarial, antitumor, antiviral, and antibacterial activities.
Medicine: It has been studied for its potential use in treating malaria, cancer, and viral infections.
Wirkmechanismus
The mechanism of action of cinchonine hydrochloride involves its interaction with molecular targets such as enzymes and receptors. It is believed to inhibit the polymerization of toxic hematin in malaria parasites, thereby exerting its antimalarial effects . Additionally, it acts as a glucagon-like peptide-1 receptor agonist, which may contribute to its potential use in treating metabolic disorders .
Vergleich Mit ähnlichen Verbindungen
Cinchonine hydrochloride is structurally similar to other Cinchona alkaloids such as quinine, quinidine, and cinchonidine . it is unique in its lower toxicity and broader spectrum of biological activities . Similar compounds include:
Quinine: Primarily used as an antimalarial agent.
Quinidine: Used as an antiarrhythmic drug.
Cinchonidine: Similar to cinchonine but with different stereochemistry.
Eigenschaften
CAS-Nummer |
5949-11-1 |
---|---|
Molekularformel |
C19H23ClN2O |
Molekulargewicht |
330.8 g/mol |
IUPAC-Name |
(S)-[(2R,4S,5S)-5-ethenyl-1-azabicyclo[2.2.2]octan-2-yl]-quinolin-4-ylmethanol;hydrochloride |
InChI |
InChI=1S/C19H22N2O.ClH/c1-2-13-12-21-10-8-14(13)11-18(21)19(22)16-7-9-20-17-6-4-3-5-15(16)17;/h2-7,9,13-14,18-19,22H,1,8,10-12H2;1H/t13-,14+,18-,19+;/m1./s1 |
InChI-Schlüssel |
IMUHWLVEEVGMBC-PAAXXVDQSA-N |
SMILES |
C=CC1CN2CCC1CC2C(C3=CC=NC4=CC=CC=C34)O.Cl |
Isomerische SMILES |
C=C[C@@H]1CN2CC[C@H]1C[C@@H]2[C@H](C3=CC=NC4=CC=CC=C34)O.Cl |
Kanonische SMILES |
C=CC1CN2CCC1CC2C(C3=CC=NC4=CC=CC=C34)O.Cl |
24302-67-8 | |
Piktogramme |
Irritant |
Herkunft des Produkts |
United States |
Retrosynthesis Analysis
AI-Powered Synthesis Planning: Our tool employs the Template_relevance Pistachio, Template_relevance Bkms_metabolic, Template_relevance Pistachio_ringbreaker, Template_relevance Reaxys, Template_relevance Reaxys_biocatalysis model, leveraging a vast database of chemical reactions to predict feasible synthetic routes.
One-Step Synthesis Focus: Specifically designed for one-step synthesis, it provides concise and direct routes for your target compounds, streamlining the synthesis process.
Accurate Predictions: Utilizing the extensive PISTACHIO, BKMS_METABOLIC, PISTACHIO_RINGBREAKER, REAXYS, REAXYS_BIOCATALYSIS database, our tool offers high-accuracy predictions, reflecting the latest in chemical research and data.
Strategy Settings
Precursor scoring | Relevance Heuristic |
---|---|
Min. plausibility | 0.01 |
Model | Template_relevance |
Template Set | Pistachio/Bkms_metabolic/Pistachio_ringbreaker/Reaxys/Reaxys_biocatalysis |
Top-N result to add to graph | 6 |
Feasible Synthetic Routes
Q1: How does cinchonine hydrochloride interact with trisoxalatometallate(III) complexes, and what are the observed effects?
A: this compound interacts with trisoxalatometallate(III) complexes through a phenomenon called the Pfeiffer effect [, ]. This effect manifests as a change in the optical rotation of the solution containing the complex upon the addition of the chiral compound, this compound.
- Association: A direct association occurs between the complex ion and the this compound molecule in solution. This is observed with the trisoxalatometallate(III) complex of Iridium(III) [].
- Association and Equilibrium Shift: In addition to the association, an "equilibrium shift" occurs between the two enantiomers of the complex in solution, favoring one over the other. This is observed with trisoxalatometallate(III) complexes of Aluminum, Iron, Chromium, and Cobalt [].
Q2: Does the presence of other solvents influence the Pfeiffer effect observed between this compound and trisoxalatometallate(III) complexes?
A: Yes, the presence of other solvents can influence the Pfeiffer effect. Research shows that lower alcohols like methanol, ethanol, and 1-propanol impact the Pfeiffer effect in systems containing tris(1,10-phenanthroline)zinc(II) sulfate with either this compound or l-strychnine hydrosulfate in water []. This suggests that solvent properties play a role in mediating the interactions between the chiral molecules and the metal complexes, ultimately affecting the observed changes in optical rotation.
Haftungsausschluss und Informationen zu In-Vitro-Forschungsprodukten
Bitte beachten Sie, dass alle Artikel und Produktinformationen, die auf BenchChem präsentiert werden, ausschließlich zu Informationszwecken bestimmt sind. Die auf BenchChem zum Kauf angebotenen Produkte sind speziell für In-vitro-Studien konzipiert, die außerhalb lebender Organismen durchgeführt werden. In-vitro-Studien, abgeleitet von dem lateinischen Begriff "in Glas", beinhalten Experimente, die in kontrollierten Laborumgebungen unter Verwendung von Zellen oder Geweben durchgeführt werden. Es ist wichtig zu beachten, dass diese Produkte nicht als Arzneimittel oder Medikamente eingestuft sind und keine Zulassung der FDA für die Vorbeugung, Behandlung oder Heilung von medizinischen Zuständen, Beschwerden oder Krankheiten erhalten haben. Wir müssen betonen, dass jede Form der körperlichen Einführung dieser Produkte in Menschen oder Tiere gesetzlich strikt untersagt ist. Es ist unerlässlich, sich an diese Richtlinien zu halten, um die Einhaltung rechtlicher und ethischer Standards in Forschung und Experiment zu gewährleisten.