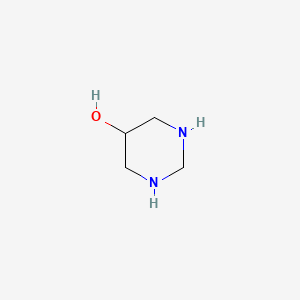
Hexahydropyrimidin-5-ol
- Klicken Sie auf QUICK INQUIRY, um ein Angebot von unserem Expertenteam zu erhalten.
- Mit qualitativ hochwertigen Produkten zu einem WETTBEWERBSFÄHIGEN Preis können Sie sich mehr auf Ihre Forschung konzentrieren.
Übersicht
Beschreibung
Hexahydropyrimidin-5-ol is a useful research compound. Its molecular formula is C4H10N2O and its molecular weight is 102.14 g/mol. The purity is usually 95%.
BenchChem offers high-quality this compound suitable for many research applications. Different packaging options are available to accommodate customers' requirements. Please inquire for more information about this compound including the price, delivery time, and more detailed information at info@benchchem.com.
Wissenschaftliche Forschungsanwendungen
Medicinal Chemistry
Antifungal Activity
Research has demonstrated that hexahydropyrimidine derivatives exhibit promising antifungal properties. A study evaluated four derivatives against common dermatomycosis fungi, including Trichophyton mentagrophytes and Microsporum canis. Among the tested compounds, one derivative (HHP3) showed the best antifungal activity with a minimum inhibitory concentration (MIC) range of 500–1000 μg/mL, indicating its potential as a therapeutic agent for fungal infections .
Synthesis of Derivatives
The synthesis of hexahydropyrimidin-5-ol derivatives has been explored to enhance their biological activities. For example, a study synthesized multiple derivatives through a Mannich-type reaction involving 1,3-diaminopropan-2-ol and paraformaldehyde. The conformational analysis revealed that the introduction of hydroxyl groups significantly alters the stereoelectronic relationships within the molecule, potentially impacting its biological activity .
Nanotechnology
Nanoparticle Engineering
this compound has been utilized in phenolic-enabled nanotechnology (PEN). This approach leverages the unique properties of phenolic compounds to engineer nanoparticles with controlled size and functionality for biomedical applications. The interactions between hexahydropyrimidine derivatives and other structural motifs facilitate the synthesis of nanohybrid materials suitable for drug delivery and biosensing .
Energetic Compounds
Design of Energetic Materials
Recent research has focused on developing energetic compounds based on hexahydropyrimidine frameworks. Twelve derivatives were synthesized by incorporating various explosophore groups into the hexahydropyrimidine structure. These compounds exhibited unique bonding characteristics that could be utilized in explosives or propellants, showcasing the versatility of hexahydropyrimidine in energetic applications .
Chemical Synthesis
Role in Ligand Chemistry
this compound plays a crucial role as a ligand in chemical synthesis processes. Its ability to form stable complexes enhances the reactivity and stability of nanoparticles used in diverse applications, including catalysis and sensor development. This property is vital for improving the performance of nanomaterials in various industrial applications .
Summary of Research Findings
Analyse Chemischer Reaktionen
Fe(III)-Catalyzed Tandem Amidomethylation
Fe(OTf)₃ catalyzes the synthesis of hexahydropyrimidine derivatives via a five-step mechanism :
- Tosylformaldimine release from bis(tosylamido)methane.
- Intermolecular aza-Prins reaction with α-phenylstyrene.
- Condensation and iminium homologation .
- Intramolecular aza-Prins cyclization to form the hexahydropyrimidine core .
Key Findings :
- The condensation/intramolecular aza-Prins pathway is favored (ΔG‡ = 1.0 kcal/mol) over alternative routes due to lower activation energies .
- Fe(OTf)₃ stabilizes intermediates via coordination, enabling high regioselectivity .
Hydrogen Bonding and Conformational Effects
The hydroxyl group at C5 induces intramolecular hydrogen bonding with adjacent nitrogen atoms, locking the hexahydropyrimidine ring into an equatorial-equatorial (ee) conformation . This effect is evident in NMR studies:
- H-5 protons resonate at ~4.00 ppm (vs. 3.57 ppm in non-hydroxylated analogs) .
- Diastereotopic CH₂ protons exhibit distinct shifts (Δ = 0.23–0.42 ppm ) due to anisotropic shielding .
Table 2: NMR Chemical Shifts for Selected Protons (CDCl₃)
Proton Position | δ (ppm) in 2a | δ (ppm) in 3 (non-hydroxylated) | Reference |
---|---|---|---|
H-5 | 4.00 | 3.57 | |
CH₂-4ax | 2.65 | 2.87 | |
CH₂-4eq | 2.88 | 3.07 |
Thermal Stability and Decomposition
This compound derivatives exhibit high thermal stability , with decomposition temperatures (TGA) ranging from 200–250°C . For instance:
- 2,2-Di(pyridin-2-yl)hexahydropyrimidine decomposes at 240°C via a single-step mechanism .
- Methoxy and tert-butyl substituents enhance stability by ~20°C compared to nitro-substituted analogs .
Mechanistic Insights from DFT Studies
Density functional theory (DFT) calculations reveal:
Q & A
Basic Research Questions
Q. What synthetic methodologies are commonly employed for preparing hexahydropyrimidin-5-ol derivatives?
this compound derivatives are typically synthesized via a condensation reaction involving 1,3-diaminopropan-2-ol, paraformaldehyde (3 equivalents), and phenolic derivatives under reflux in methanol. The reaction time varies depending on the substituents, as shown in Table 1 of . Post-reaction, the product is isolated by filtration, washed with cold ethanol, and dried in vacuo. This method emphasizes stoichiometric control and solvent selection to optimize yield .
Q. How does NMR spectroscopy aid in distinguishing axial vs. equatorial hydroxyl configurations in this compound derivatives?
Axial hydroxyl groups in this compound derivatives exhibit a characteristic coupling constant (2J = 12 Hz) in 1H-NMR, observed in CDCl₃. For example, H-5 protons in compounds with axial hydroxyls resonate at ~4.00 ppm, compared to ~3.57 ppm in derivatives without intramolecular hydrogen bonding. Diastereotopic methylene protons adjacent to the hydroxyl group also show downfield shifts (e.g., 2.65–3.07 ppm), providing diagnostic structural insights .
Q. What analytical techniques are critical for confirming the purity and identity of this compound derivatives?
Key techniques include:
- NMR Spectroscopy : To resolve hydrogen bonding effects and ring inversion dynamics (e.g., slow inversion on the NMR timescale causes split signals).
- X-ray Crystallography : For unambiguous confirmation of hydrogen-bonded networks, as demonstrated in the crystal structure of 1,3-bis(3-tert-butyl-2-hydroxy-5-methoxybenzyl)this compound monohydrate .
- Chromatography : HPLC or TLC to verify purity, using methods like ammonium acetate buffer (pH 6.5) for separation .
Advanced Research Questions
Q. How do intramolecular hydrogen bonds affect the stability and spectroscopic properties of this compound derivatives?
Intramolecular hydrogen bonds between the hydroxyl group and phenolic substituents stabilize the axial conformation, as shown by 1H-NMR data. This stabilization slows ring inversion, leading to broader axial proton signals. The deshielding effect on adjacent methylene protons (e.g., shifts from 2.65 ppm to 3.07 ppm) further confirms hydrogen bonding, which can be disrupted by polar solvents or temperature variations .
Q. What experimental strategies resolve contradictions in reported NMR chemical shifts for this compound derivatives?
Contradictions may arise from solvent polarity, temperature, or substituent effects. To address this:
- Use control compounds (e.g., this compound (3) in ) for comparative analysis.
- Standardize solvent conditions (e.g., CDCl₃ vs. DMSO-d₆) and temperature during NMR acquisition.
- Employ 2D NMR (e.g., COSY, NOESY) to resolve overlapping signals caused by slow ring inversion .
Q. How can reaction conditions be optimized to improve the yield of this compound derivatives?
Optimization strategies include:
- Paraformaldehyde Equivalents : Increasing from 3 to 4 equivalents to drive the condensation reaction to completion.
- Reaction Time : Extending reflux duration (e.g., 12–24 hours) for sterically hindered phenolic substrates.
- Solvent Screening : Testing aprotic solvents (e.g., THF) to minimize side reactions .
Q. How can computational methods complement experimental data in studying this compound derivatives?
Density Functional Theory (DFT) calculations (e.g., Gaussian 09) can model hydrogen-bond strengths, ring inversion barriers, and electronic environments. These simulations align with experimental NMR shifts and predict solvent effects, aiding in mechanistic studies .
Q. Methodological Notes
- Spectral Interpretation : Always compare experimental NMR data with reference compounds to account for solvent- or substituent-induced shifts.
- Synthesis Reproducibility : Document paraformaldehyde purity and stoichiometry, as deviations can lead to incomplete ring formation.
- Safety Protocols : Handle derivatives in fume hoods to avoid inhalation risks, especially during solvent reflux and filtration steps .
Eigenschaften
Molekularformel |
C4H10N2O |
---|---|
Molekulargewicht |
102.14 g/mol |
IUPAC-Name |
1,3-diazinan-5-ol |
InChI |
InChI=1S/C4H10N2O/c7-4-1-5-3-6-2-4/h4-7H,1-3H2 |
InChI-Schlüssel |
ROYZEMODCVMWJX-UHFFFAOYSA-N |
Kanonische SMILES |
C1C(CNCN1)O |
Herkunft des Produkts |
United States |
Haftungsausschluss und Informationen zu In-Vitro-Forschungsprodukten
Bitte beachten Sie, dass alle Artikel und Produktinformationen, die auf BenchChem präsentiert werden, ausschließlich zu Informationszwecken bestimmt sind. Die auf BenchChem zum Kauf angebotenen Produkte sind speziell für In-vitro-Studien konzipiert, die außerhalb lebender Organismen durchgeführt werden. In-vitro-Studien, abgeleitet von dem lateinischen Begriff "in Glas", beinhalten Experimente, die in kontrollierten Laborumgebungen unter Verwendung von Zellen oder Geweben durchgeführt werden. Es ist wichtig zu beachten, dass diese Produkte nicht als Arzneimittel oder Medikamente eingestuft sind und keine Zulassung der FDA für die Vorbeugung, Behandlung oder Heilung von medizinischen Zuständen, Beschwerden oder Krankheiten erhalten haben. Wir müssen betonen, dass jede Form der körperlichen Einführung dieser Produkte in Menschen oder Tiere gesetzlich strikt untersagt ist. Es ist unerlässlich, sich an diese Richtlinien zu halten, um die Einhaltung rechtlicher und ethischer Standards in Forschung und Experiment zu gewährleisten.