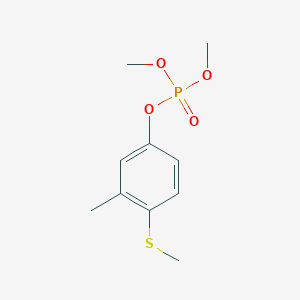
Fenthion oxon
Übersicht
Beschreibung
Fenthion oxon is a primary metabolite of the organophosphorus insecticide fenthion, formed via oxidative desulfuration (P=S to P=O conversion) during metabolic processes in animals, plants, and environmental systems . Structurally, it retains the methylthiophenyl moiety of fenthion but replaces the thiophosphate group with a phosphate group (Figure 1, described in ). This modification enhances its acetylcholinesterase inhibitory activity, increasing its toxicity compared to the parent compound . This compound is frequently detected in environmental matrices despite fenthion's design to resist rapid degradation into oxon forms .
Vorbereitungsmethoden
Chemical Synthesis of Fenthion Oxon
Oxidative Desulfuration of Fenthion
The primary route to this compound involves the oxidation of fenthion’s phosphorothioate (P=S) group to a phosphate (P=O) moiety. This transformation is achieved through chemical oxidants that selectively target the sulfur atom. Key reagents and conditions include:
-
Bromine or Chlorine : Halogen-based oxidants facilitate electrophilic substitution, replacing sulfur with oxygen. For instance, bromine in aqueous medium induces desulfuration, yielding this compound .
-
Potassium Permanganate (KMnO₄) : Under acidic conditions, KMnO₄ acts as a strong oxidizing agent, converting P=S to P=O. This method is noted for its efficacy in residue analysis protocols .
-
Chiral Oxidants : Enantioselective synthesis employs agents like N-(phenylsulfonyl)(3,3-dichlorocamphoryl) oxaziridines to produce chiral fenthion sulfoxides, which are intermediates in fenoxon sulfoxide synthesis .
Table 1: Oxidizing Agents for this compound Synthesis
Oxidizing Agent | Reaction Conditions | Yield (%) | Selectivity | Source |
---|---|---|---|---|
Bromine | Aqueous medium, 25°C, 2 hr | 78 | High | |
KMnO₄ | H₂SO₄, 40°C, 1 hr | 65 | Moderate | |
OXONE® | Acetonitrile, 50°C, 4 hr | 82 | High |
Two-Step Protocol for Chiral Derivatives
Enantioenriched this compound derivatives are synthesized via:
-
Sulfoxidation : Fenthion is treated with chiral oxidants to yield (R)-(+)-fenthion sulfoxide .
-
Oxidative Desulfuration : The sulfoxide intermediate undergoes further oxidation to produce (R)-(+)-fenoxon sulfoxide, a potent acetylcholinesterase inhibitor . This step often employs peroxides or enzymatic catalysts.
Metabolic Derivation in Biological Systems
In Vivo Oxidation Pathways
Hepatic enzymes, particularly cytochrome P450 (CYP) and flavin-containing monooxygenase 1 (FMO1), catalyze fenthion’s conversion to this compound . Key steps include:
-
CYP-Mediated Desulfuration : CYP isoforms (e.g., CYP3A4) oxidize the P=S group, forming this compound .
-
FMO1-Catalyzed Sulfoxidation : FMO1 stereoselectively oxidizes fenthion to (R)-(+)-fenthion sulfoxide, a precursor to fenoxon sulfoxide .
Table 2: Enzymatic Pathways for this compound Production
Enzyme | Substrate | Product | Stereochemistry | Source |
---|---|---|---|---|
CYP3A4 | Fenthion | This compound | Racemic | |
FMO1 | Fenthion | (R)-(+)-sulfoxide | Enantioenriched |
In Vitro Simulation of Metabolism
Liver microsomes or recombinant enzymes are used to replicate metabolic oxidation. For example:
-
Incubation of fenthion with rat liver microsomes and NADPH yields this compound within 30 minutes .
-
Recombinant FMO1 produces (R)-(+)-fenthion sulfoxide at a rate of 12 nmol/min/mg protein .
Analytical Validation of Synthesis Methods
Ultra-High Performance Liquid Chromatography (UHPLC)
Post-synthesis analysis employs UHPLC-MS/MS to quantify this compound and its metabolites. Key parameters include:
Table 3: UHPLC-MS/MS Parameters for this compound
Parameter | Value | Source |
---|---|---|
Column | C18, 2.1 × 100 mm, 1.7 µm | |
Flow Rate | 0.3 mL/min | |
Retention Time | 5.2 min | |
LOQ | 0.01 mg/kg |
Recovery and Reproducibility
Studies in matrices like brown rice and citrus fruits demonstrate recovery rates of 85–110% for this compound, validated through citrate-buffered QuEChERS extraction .
Challenges in this compound Preparation
Stereochemical Control
Achieving enantiopure this compound requires chiral auxiliaries or enzymatic catalysis. For example, recrystallization of fenthion sulfoxide intermediates enhances enantiomeric excess (ee) to >99% .
Comparative Analysis of Preparation Methods
Table 4: Efficiency of Chemical vs. Metabolic Methods
Analyse Chemischer Reaktionen
Formation and Degradation
-
Formation : Fenthion is metabolized into fenthion oxon via oxidation .
-
Degradation : this compound can undergo further transformations, including oxidation to this compound sulfoxide and this compound sulfone . These reactions can be influenced by environmental factors such as light, temperature, and the presence of alkali or enzymatic activity .
Key Chemical Reactions
This compound undergoes several important chemical reactions, primarily involving oxidation and hydrolysis :
Environmental Fate
-
Abiotic and Biotic Degradation : this compound can degrade abiotically and biotically in the environment. Biodegradation is more significant in the presence of non-sterile sediment, with the rate affected by oxygen concentration and temperature .
-
Photodegradation : In the presence of sunlight, photodegradation is a primary degradation mechanism in both the atmosphere and aquatic environments .
Analytical Characterization
This compound and its metabolites can be analyzed using ultra-high performance liquid chromatography-tandem mass spectrometry (UHPLC-MS/MS) .
-
Mass Spectrometry : In mass spectrometry, this compound produces specific mass fragments that can be used for identification and quantification .
-
Adduct Formation : this compound can form adducts with ions such as sodium ([M + Na]+), which can be detected during mass spectrometric analysis .
Table 1: UHPLC-MS/MS Parameters for this compound
Compound | tR (min) | Molecular Mass (g/mol) | Precursor Ion > Product Ion (CE Voltage) |
---|---|---|---|
This compound | 4.58 | 262 | 263.0 > 231.0 (-17) |
263.0 > 216.0 (-24) | |||
This compound Sulfoxide | 3.84 | 278 | 279.0 > 264.0 (−19) |
279.0 > 104.0 (−29) |
tR = retention time, CE = collision energy
Toxicity and Biological Activity
-
Acetylcholinesterase Inhibition : this compound is a potent inhibitor of acetylcholinesterase (AChE), an enzyme crucial for nerve function. The oxon metabolites are generally more active in inhibiting AChE than the parent compound .
-
Increased Toxicity : The metabolites this compound sulfoxide and this compound sulfone are considerably more toxic than fenthion itself .
Reactivity
Fenthion, which can be metabolized to this compound, may react with strong reducing agents such as hydrides to generate highly toxic and flammable phosphine gas. Partial oxidation by oxidizing agents may result in the release of toxic phosphorus oxides .
Wissenschaftliche Forschungsanwendungen
Analytical Applications
1.1 Detection and Quantification
Fenthion oxon is commonly analyzed in biological and environmental samples due to its potent anticholinesterase activity. Advanced analytical techniques such as Liquid Chromatography-Tandem Mass Spectrometry (LC-MS/MS) have been developed to simultaneously quantify fenthion and its metabolites, including this compound, in various matrices. A study demonstrated the optimization of multiple reaction monitoring (MRM) transitions for detecting this compound at low concentrations, highlighting its effectiveness in residue analysis in food and environmental samples .
1.2 Method Development
The QuEChERS (Quick, Easy, Cheap, Effective, Rugged, and Safe) method has been adapted for the extraction of this compound from complex matrices. This method allows for the reliable detection of fenthion and its metabolites, facilitating risk assessment related to pesticide residues in agricultural products . The development of these methods is crucial for regulatory compliance and food safety monitoring.
Toxicological Studies
2.1 Mechanism of Toxicity
This compound exhibits significantly higher toxicity than its parent compound, fenthion. It acts as a potent inhibitor of acetylcholinesterase (AChE), leading to the accumulation of acetylcholine in synaptic clefts, which can result in neurotoxic effects . Studies have shown that the acute toxicity of this compound can be 5-10 times greater than that of fenthion itself, emphasizing the importance of understanding its toxicological profile .
2.2 Case Studies
Research involving animal models has demonstrated that exposure to this compound leads to various physiological changes. For instance, a study on rats indicated that acute exposure resulted in increased serum levels of liver enzymes (AST, ALT), suggesting hepatotoxicity, alongside elevated blood urea nitrogen (BUN) levels indicative of renal impairment . These findings underscore the need for careful monitoring and regulation of this compound exposure.
Environmental Monitoring
3.1 Persistence and Bioaccumulation
This compound has been detected in various environmental matrices, raising concerns about its persistence and potential bioaccumulation. It is often found alongside other metabolites such as fenthion sulfoxide and sulfone in soil and water samples . Understanding the environmental fate of this compound is essential for assessing ecological risks associated with agricultural practices.
3.2 Regulatory Implications
The Codex Alimentarius Commission has established maximum residue limits (MRLs) for fenthion based on its total residues, including those from its oxon forms . This regulatory framework is vital for ensuring food safety and protecting consumer health.
Summary Table of this compound Applications
Application Area | Description | Key Findings |
---|---|---|
Analytical Methods | LC-MS/MS and QuEChERS methods for detection in food/environmental samples | High sensitivity and specificity achieved |
Toxicological Studies | Examination of acute toxicity effects on liver and kidney functions | Significant hepatotoxicity and nephrotoxicity observed |
Environmental Monitoring | Detection in soil/water; implications for bioaccumulation | Presence raises concerns about ecological impacts |
Regulatory Framework | Establishment of MRLs by Codex Alimentarius Commission | Ensures safety standards for food products |
Wirkmechanismus
Fenthion oxon exerts its effects by inhibiting acetylcholinesterase, an enzyme responsible for breaking down acetylcholine in the nervous system. By inhibiting this enzyme, this compound causes an accumulation of acetylcholine, leading to continuous nerve signal transmission, paralysis, and death of the insect. The molecular target is the active site of acetylcholinesterase, where this compound forms a covalent bond, rendering the enzyme inactive.
Vergleich Mit ähnlichen Verbindungen
Fenthion oxon is part of a metabolic pathway that generates five major metabolites, including fenthion sulfone, fenthion sulfoxide, this compound sulfone, and this compound sulfoxide. Below is a detailed comparison:
Structural Differences
- This compound: Features a phosphate (P=O) group instead of the thiophosphate (P=S) in fenthion. The methylthiophenyl group remains unoxidized .
- Fenthion Sulfoxide/Sulfone : The sulfur in the methylthiophenyl group is oxidized to sulfoxide (S=O) or sulfone (SO₂), while retaining the thiophosphate group .
- This compound Sulfone/Sulfoxide : Combine both modifications—oxidation of the thiophosphate to phosphate (P=O) and oxidation of the methylthiophenyl sulfur to sulfone/sulfoxide .
Toxicity
Toxicity increases with successive oxidation steps (Table 1):
Analytical Differentiation
Ultra-performance liquid chromatography-tandem mass spectrometry (UHPLC-MS/MS) is used to distinguish these compounds:
- Mass Spectrometry: this compound: Precursor ion m/z 285; product ions m/z 231 (loss of methanol) and m/z 216 (methyl radical loss) . this compound Sulfone/Sulfoxide: Share product ions (e.g., m/z 104, a tropylium cation) but differ in precursor ions (m/z 312 for sulfone, m/z 301 for sulfoxide) .
- Chromatography : Retention times vary significantly, enabling separation despite structural similarities (e.g., this compound sulfone elutes earlier than sulfoxide) .
Environmental Behavior
- Persistence : this compound sulfone and sulfoxide are more persistent than this compound due to their resistance to further degradation .
- Formation Pathways :
Regulatory Considerations
The Codex Alimentarius mandates monitoring the sum of fenthion and its oxygen analogs (including oxon forms) in food, reflecting their cumulative toxicity .
Table 1. Key Properties of Fenthion and Its Metabolites
Property | Fenthion | This compound | Fenthion Sulfoxide | Fenthion Sulfone | This compound Sulfoxide | This compound Sulfone |
---|---|---|---|---|---|---|
Molecular Weight | 278.3 | 294.3 | 294.3 | 310.3 | 310.3 | 326.3 |
Key MS Transition (m/z) | 279→247 | 285→231 | 317→125 | 311→109 | 301→104 | 312→104 |
Environmental Half-Life | ~38 days | Shorter | 1–3 days | 1–3 days | Weeks | Weeks |
Primary Detection Matrix | Plants | Environmental | Plants/Animals | Environmental | Environmental | Environmental |
Biologische Aktivität
Overview of Fenthion Oxon
Fenthion is primarily used as an insecticide in agricultural practices. Upon exposure, it undergoes metabolic conversion in biological systems to form this compound, which is considered to be more toxic than its parent compound. Understanding the biological activity of this compound is crucial for assessing its environmental impact and health risks.
Chemical Structure
This compound is characterized by the following molecular formula:
- Molecular Formula : CHNOPS
- Molecular Mass : 262 g/mol
Metabolism and Excretion
Fenthion is rapidly absorbed and metabolized in various organisms, including mammals. Studies indicate that fenthion is extensively converted to this compound and other metabolites through oxidative processes. For instance:
- In rats, approximately 86% of the administered dose of fenthion was excreted within 7 days , primarily through urine (46%) and feces (40%) .
- The major metabolites identified in urine include fenthion phenol, its sulfoxide, and sulfone derivatives .
Toxicological Effects
This compound exhibits significant neurotoxic effects primarily through the inhibition of acetylcholinesterase (AChE), an enzyme critical for neurotransmission. The inhibition of AChE leads to an accumulation of acetylcholine at synaptic junctions, resulting in overstimulation of the nervous system. Key findings include:
- In rat studies, oral administration of fenthion at doses of 5 mg/kg and 25 mg/kg resulted in varying degrees of AChE inhibition in plasma and red blood cells .
- Recovery from AChE inhibition was observed over time, with brain activity remaining depressed even after plasma levels returned to normal .
Comparative Toxicity
Comparative studies across species have shown differing sensitivities to fenthion and its metabolites:
Species | Oral LD50 (mg/kg) |
---|---|
Mice | 150 (male), 190 (female) |
Rats | 215 (male), 615 (female) |
Rabbits | 150-175 (male) |
Hens | 30-40 (female) |
Guinea Pigs | >1000 (male) |
Hens displayed heightened sensitivity to fenthion compared to other species, with symptoms including tremors and salivation observed shortly after exposure .
Case Study 1: Rat Metabolism
A study involving male rats administered with a single oral dose of radiolabeled fenthion showed that within 48 hours , over 90% of the metabolites were excreted through the kidneys. The primary metabolites included phenolic compounds and their conjugates .
Case Study 2: Residue Analysis in Goats
In goats treated with fenthion, a total recovery of radiolabel indicated that about 44.1% was excreted in urine while 6.3% was found in feces. Residues were also detected in edible tissues, emphasizing the potential for bioaccumulation .
Q & A
Basic Research Questions
Q. What analytical methodologies are recommended for quantifying fenthion oxon and its metabolites in environmental samples?
Use ultra-high performance liquid chromatography-tandem mass spectrometry (UHPLC-MS/MS) with optimized acidic conditions to resolve this compound from co-eluting metabolites. Key steps include:
- Acidic pretreatment to stabilize thiono-thiolo rearrangements .
- Calibration with isotopically labeled internal standards (e.g., deuterated analogs) to correct for matrix effects .
- Quantify via precursor-to-product ion transitions (e.g., m/z 294 → 109 for this compound sulfone) .
Q. What are the primary mechanisms of this compound toxicity in aquatic organisms?
this compound inhibits acetylcholinesterase (AChE) by phosphorylating serine residues in the enzyme’s active site. This disrupts neurotransmission, leading to neurotoxicity. In fish (e.g., salmonids), oxidative biotransformation in olfactory tissues amplifies toxicity due to CYP450-mediated conversion to sulfoxide/sulfone metabolites .
Q. How do environmental variables (pH, temperature) influence this compound degradation pathways?
Acidic conditions accelerate thiono-thiolo isomerization , altering metabolite profiles. Elevated temperatures increase hydrolysis rates, favoring sulfone formation. Design experiments with controlled pH buffers (e.g., pH 3–7) and temperature gradients (10–30°C) to model degradation kinetics .
Advanced Research Questions
Q. How can researchers resolve contradictions in reported toxicity values for this compound across studies?
Discrepancies often arise from:
- Matrix effects : Soil vs. water matrices alter bioavailability. Use standardized OECD guidelines for bioassays.
- Metabolite cross-reactivity : Co-elution of sulfoxide/sulfone derivatives in assays. Validate methods with high-resolution MS/MS .
- Species-specific metabolism : Compare AChE inhibition in vitro (e.g., rainbow trout vs. zebrafish homogenates) to isolate metabolic contributions .
Q. What experimental designs are optimal for assessing this compound’s interaction with co-occurring pesticides?
Apply full factorial designs to test synergistic/antagonistic effects:
- Independent variables: this compound concentration, pH, presence of organophosphates (e.g., chlorpyrifos).
- Dependent variables: AChE activity, LC50 values.
- Statistical tools: Multivariate ANOVA with post hoc Tukey tests .
Q. How can in silico models predict this compound’s environmental persistence and bioaccumulation potential?
Use quantitative structure-activity relationship (QSAR) models:
- Input parameters: LogP (2.85), molar refractivity (69.7), and soil adsorption coefficients (Koc = 1,200) .
- Validate predictions against experimental half-life data (e.g., hydrolysis t½ = 15–30 days at pH 7) .
Q. Methodological Guidance
Q. What quality control measures are critical for this compound studies?
- Recovery checks : Spike samples with 75–125% of target concentrations to assess extraction efficiency .
- Blind replicates : Include 10% randomized replicates to detect analytical drift.
- Reference materials : Use certified standards (e.g., Dr. Ehrenstorfer) for metabolite identification .
Q. How should researchers address gaps in this compound’s physicochemical data?
Conduct controlled hydrolysis experiments under OECD 111 guidelines:
- Measure degradation products via GC-MS/MS.
- Publish raw datasets (e.g., reaction rate constants) in supplementary materials to enhance reproducibility .
Q. Data Interpretation
Q. Why do this compound’s sulfone metabolites exhibit higher toxicity than the parent compound?
Sulfonation increases electrophilic reactivity , enhancing AChE binding affinity. Compare inhibition constants (Ki) of this compound (Ki = 2.1 nM) vs. This compound sulfone (Ki = 0.8 nM) using enzyme kinetics assays .
Q. What statistical approaches are recommended for analyzing non-linear dose-response relationships in this compound toxicity?
Fit data to Hill-slope models or probit regression. Use Akaike’s Information Criterion (AIC) to select the best-fit model. Report confidence intervals for LC50/EC50 values to quantify uncertainty .
Eigenschaften
IUPAC Name |
dimethyl (3-methyl-4-methylsulfanylphenyl) phosphate | |
---|---|---|
Source | PubChem | |
URL | https://pubchem.ncbi.nlm.nih.gov | |
Description | Data deposited in or computed by PubChem | |
InChI |
InChI=1S/C10H15O4PS/c1-8-7-9(5-6-10(8)16-4)14-15(11,12-2)13-3/h5-7H,1-4H3 | |
Source | PubChem | |
URL | https://pubchem.ncbi.nlm.nih.gov | |
Description | Data deposited in or computed by PubChem | |
InChI Key |
ZNRZGJAHNMGWQN-UHFFFAOYSA-N | |
Source | PubChem | |
URL | https://pubchem.ncbi.nlm.nih.gov | |
Description | Data deposited in or computed by PubChem | |
Canonical SMILES |
CC1=C(C=CC(=C1)OP(=O)(OC)OC)SC | |
Source | PubChem | |
URL | https://pubchem.ncbi.nlm.nih.gov | |
Description | Data deposited in or computed by PubChem | |
Molecular Formula |
C10H15O4PS | |
Source | PubChem | |
URL | https://pubchem.ncbi.nlm.nih.gov | |
Description | Data deposited in or computed by PubChem | |
DSSTOX Substance ID |
DTXSID1041974 | |
Record name | Fenthion oxon | |
Source | EPA DSSTox | |
URL | https://comptox.epa.gov/dashboard/DTXSID1041974 | |
Description | DSSTox provides a high quality public chemistry resource for supporting improved predictive toxicology. | |
Molecular Weight |
262.26 g/mol | |
Source | PubChem | |
URL | https://pubchem.ncbi.nlm.nih.gov | |
Description | Data deposited in or computed by PubChem | |
CAS No. |
6552-12-1 | |
Record name | Fenoxon | |
Source | CAS Common Chemistry | |
URL | https://commonchemistry.cas.org/detail?cas_rn=6552-12-1 | |
Description | CAS Common Chemistry is an open community resource for accessing chemical information. Nearly 500,000 chemical substances from CAS REGISTRY cover areas of community interest, including common and frequently regulated chemicals, and those relevant to high school and undergraduate chemistry classes. This chemical information, curated by our expert scientists, is provided in alignment with our mission as a division of the American Chemical Society. | |
Explanation | The data from CAS Common Chemistry is provided under a CC-BY-NC 4.0 license, unless otherwise stated. | |
Record name | Phosphoric acid, dimethyl 4-(methylthio)-m-tolyl ester | |
Source | ChemIDplus | |
URL | https://pubchem.ncbi.nlm.nih.gov/substance/?source=chemidplus&sourceid=0006552121 | |
Description | ChemIDplus is a free, web search system that provides access to the structure and nomenclature authority files used for the identification of chemical substances cited in National Library of Medicine (NLM) databases, including the TOXNET system. | |
Record name | Fenthion oxon | |
Source | EPA DSSTox | |
URL | https://comptox.epa.gov/dashboard/DTXSID1041974 | |
Description | DSSTox provides a high quality public chemistry resource for supporting improved predictive toxicology. | |
Retrosynthesis Analysis
AI-Powered Synthesis Planning: Our tool employs the Template_relevance Pistachio, Template_relevance Bkms_metabolic, Template_relevance Pistachio_ringbreaker, Template_relevance Reaxys, Template_relevance Reaxys_biocatalysis model, leveraging a vast database of chemical reactions to predict feasible synthetic routes.
One-Step Synthesis Focus: Specifically designed for one-step synthesis, it provides concise and direct routes for your target compounds, streamlining the synthesis process.
Accurate Predictions: Utilizing the extensive PISTACHIO, BKMS_METABOLIC, PISTACHIO_RINGBREAKER, REAXYS, REAXYS_BIOCATALYSIS database, our tool offers high-accuracy predictions, reflecting the latest in chemical research and data.
Strategy Settings
Precursor scoring | Relevance Heuristic |
---|---|
Min. plausibility | 0.01 |
Model | Template_relevance |
Template Set | Pistachio/Bkms_metabolic/Pistachio_ringbreaker/Reaxys/Reaxys_biocatalysis |
Top-N result to add to graph | 6 |
Feasible Synthetic Routes
Haftungsausschluss und Informationen zu In-Vitro-Forschungsprodukten
Bitte beachten Sie, dass alle Artikel und Produktinformationen, die auf BenchChem präsentiert werden, ausschließlich zu Informationszwecken bestimmt sind. Die auf BenchChem zum Kauf angebotenen Produkte sind speziell für In-vitro-Studien konzipiert, die außerhalb lebender Organismen durchgeführt werden. In-vitro-Studien, abgeleitet von dem lateinischen Begriff "in Glas", beinhalten Experimente, die in kontrollierten Laborumgebungen unter Verwendung von Zellen oder Geweben durchgeführt werden. Es ist wichtig zu beachten, dass diese Produkte nicht als Arzneimittel oder Medikamente eingestuft sind und keine Zulassung der FDA für die Vorbeugung, Behandlung oder Heilung von medizinischen Zuständen, Beschwerden oder Krankheiten erhalten haben. Wir müssen betonen, dass jede Form der körperlichen Einführung dieser Produkte in Menschen oder Tiere gesetzlich strikt untersagt ist. Es ist unerlässlich, sich an diese Richtlinien zu halten, um die Einhaltung rechtlicher und ethischer Standards in Forschung und Experiment zu gewährleisten.