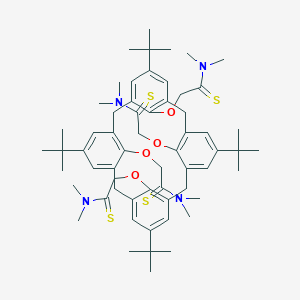
Lead ionophore IV
Übersicht
Beschreibung
Lead ionophore IV, also known as tert-Butylcalix4arene-tetrakis(N,N-dimethylthioacetamide), is a highly Pb-selective polymer for membrane electrodes . It is used in the fabrication of robust potentiometric ion sensors and as the base for the synthesis of lead-selective micro-electrodes .
Synthesis Analysis
This compound is used in the fabrication of robust potentiometric ion sensors . It is also used as the base for the synthesis of lead-selective micro-electrodes . The exact synthesis process is not detailed in the available sources.Molecular Structure Analysis
The molecular weight of this compound is 1053.6 . It has a complex structure with the formula C60H84N4O4S4 . The exact molecular structure is not detailed in the available sources.Chemical Reactions Analysis
This compound is used in the fabrication of robust potentiometric ion sensors . The detection mechanism is based on the complexation of the Pb2+ ions by the lead ionophores within the membrane, thus modulating the interactions between the ionophores and the chemiresistive film .Physical and Chemical Properties Analysis
This compound has a molecular weight of 1053.6 . It has a complex structure with the formula C60H84N4O4S4 . The ionophore should be lipophilic (as opposed to hydrophilic) so that it is not leached from the membrane upon exposure to aqueous solutions .Wissenschaftliche Forschungsanwendungen
Nanomaterial/Ionophore-Based Electrode for Anodic Stripping Voltammetric Determination of Lead
A novel nanomaterial/ionophore-modified glassy carbon electrode for anodic stripping analysis of lead (Pb(2+)) has been developed. This electrode utilizes nanosized hydroxyapatite and an ionophore, resulting in remarkably improved sensitivity and selectivity to Pb(2+). It offers a linear range of 5.0 nM to 0.8 µM for Pb(2+) detection, with a sensitivity of 13 µA/µM and a detection limit of 1.0 nM. This technology is effective for trace-level determination of Pb(2+) in real water samples (Pan et al., 2009).
Carbon Nanotubes/Ionophore Modified Electrode for Anodic Stripping Determination of Lead
The combination of carbon nanotubes and ionophore has been introduced for anodic stripping analysis of lead (Pb2+). This modified electrode shows improved sensitivity and selectivity for Pb2+, with a low detection limit of 1 nM and a wide linear range (5 nM–8 µM). Its practical application has been successful in determining Pb2+ in real water samples (Lou et al., 2011).
Macrocyclic Oxamides as Ionophores for Lead-Selective Membrane Electrodes
Macrocyclic oxamides have been investigated as potential ionophores for lead ions in solvent polymeric membrane electrodes. Lead-selective electrodes using these oxamides demonstrated pronounced preference for lead over other divalent and some monovalent cations (Malinowska et al., 1993).
Lead-Selective Membrane Electrodes Based on Neutral Carriers
A group of amides and oxamides has been used as potential ionophores for lead ions in solvent polymeric membrane electrodes. The electrodes demonstrated favorable analytical results, including a linear response with a slope of 35.3 mV per pPb unit in PbII and an activity range of 10–5.2–10–1.0M (Malinowska, 1990).
Ionophore/Ionomer Films on Glassy Carbon Electrodes for Accumulation Voltammetry
This research describes the use of ionophores in voltammetry with modified glassy carbon electrodes. A lead(II) ionophore, methylenebis (N, N-diisobutyldithiocarbamate), was used, showing potential for detection of lead(II) down to 1 × 10–7 mol L–1 with a 600 s accumulation time (Arrigan et al., 1994).
Lead-Selective Bulk Optodes Based on Neutral Ionophores
This study presents an optical sensor (optode) for measuring lead, incorporating a metal ion-selective ionophore and a proton-selective chromoionophore. The optode demonstrated an excellent selectivity over alkali and alkaline-earth metals with a measuring range for lead found to be 5.0×10 -9 to 5.0×10 -3 M (Lerchi et al., 1992).
Wirkmechanismus
Zukünftige Richtungen
Lead ionophore IV has been used in the fabrication of robust potentiometric ion sensors and as the base for the synthesis of lead-selective micro-electrodes . Future research could focus on improving the sensitivity and selectivity of these sensors, as well as exploring other potential applications of this compound.
Eigenschaften
IUPAC Name |
N,N-dimethyl-2-[[5,11,17,23-tetratert-butyl-26,27,28-tris[2-(dimethylamino)-2-sulfanylideneethoxy]-25-pentacyclo[19.3.1.13,7.19,13.115,19]octacosa-1(24),3,5,7(28),9,11,13(27),15(26),16,18,21(25),22-dodecaenyl]oxy]ethanethioamide | |
---|---|---|
Source | PubChem | |
URL | https://pubchem.ncbi.nlm.nih.gov | |
Description | Data deposited in or computed by PubChem | |
InChI |
InChI=1S/C60H84N4O4S4/c1-57(2,3)45-25-37-21-39-27-46(58(4,5)6)29-41(54(39)66-34-50(70)62(15)16)23-43-31-48(60(10,11)12)32-44(56(43)68-36-52(72)64(19)20)24-42-30-47(59(7,8)9)28-40(55(42)67-35-51(71)63(17)18)22-38(26-45)53(37)65-33-49(69)61(13)14/h25-32H,21-24,33-36H2,1-20H3 | |
Source | PubChem | |
URL | https://pubchem.ncbi.nlm.nih.gov | |
Description | Data deposited in or computed by PubChem | |
InChI Key |
CDVPPFVKRDRQTO-UHFFFAOYSA-N | |
Source | PubChem | |
URL | https://pubchem.ncbi.nlm.nih.gov | |
Description | Data deposited in or computed by PubChem | |
Canonical SMILES |
CC(C)(C)C1=CC2=C(C(=C1)CC3=CC(=CC(=C3OCC(=S)N(C)C)CC4=CC(=CC(=C4OCC(=S)N(C)C)CC5=C(C(=CC(=C5)C(C)(C)C)C2)OCC(=S)N(C)C)C(C)(C)C)C(C)(C)C)OCC(=S)N(C)C | |
Source | PubChem | |
URL | https://pubchem.ncbi.nlm.nih.gov | |
Description | Data deposited in or computed by PubChem | |
Molecular Formula |
C60H84N4O4S4 | |
Source | PubChem | |
URL | https://pubchem.ncbi.nlm.nih.gov | |
Description | Data deposited in or computed by PubChem | |
Molecular Weight |
1053.6 g/mol | |
Source | PubChem | |
URL | https://pubchem.ncbi.nlm.nih.gov | |
Description | Data deposited in or computed by PubChem | |
Retrosynthesis Analysis
AI-Powered Synthesis Planning: Our tool employs the Template_relevance Pistachio, Template_relevance Bkms_metabolic, Template_relevance Pistachio_ringbreaker, Template_relevance Reaxys, Template_relevance Reaxys_biocatalysis model, leveraging a vast database of chemical reactions to predict feasible synthetic routes.
One-Step Synthesis Focus: Specifically designed for one-step synthesis, it provides concise and direct routes for your target compounds, streamlining the synthesis process.
Accurate Predictions: Utilizing the extensive PISTACHIO, BKMS_METABOLIC, PISTACHIO_RINGBREAKER, REAXYS, REAXYS_BIOCATALYSIS database, our tool offers high-accuracy predictions, reflecting the latest in chemical research and data.
Strategy Settings
Precursor scoring | Relevance Heuristic |
---|---|
Min. plausibility | 0.01 |
Model | Template_relevance |
Template Set | Pistachio/Bkms_metabolic/Pistachio_ringbreaker/Reaxys/Reaxys_biocatalysis |
Top-N result to add to graph | 6 |
Feasible Synthetic Routes
Haftungsausschluss und Informationen zu In-Vitro-Forschungsprodukten
Bitte beachten Sie, dass alle Artikel und Produktinformationen, die auf BenchChem präsentiert werden, ausschließlich zu Informationszwecken bestimmt sind. Die auf BenchChem zum Kauf angebotenen Produkte sind speziell für In-vitro-Studien konzipiert, die außerhalb lebender Organismen durchgeführt werden. In-vitro-Studien, abgeleitet von dem lateinischen Begriff "in Glas", beinhalten Experimente, die in kontrollierten Laborumgebungen unter Verwendung von Zellen oder Geweben durchgeführt werden. Es ist wichtig zu beachten, dass diese Produkte nicht als Arzneimittel oder Medikamente eingestuft sind und keine Zulassung der FDA für die Vorbeugung, Behandlung oder Heilung von medizinischen Zuständen, Beschwerden oder Krankheiten erhalten haben. Wir müssen betonen, dass jede Form der körperlichen Einführung dieser Produkte in Menschen oder Tiere gesetzlich strikt untersagt ist. Es ist unerlässlich, sich an diese Richtlinien zu halten, um die Einhaltung rechtlicher und ethischer Standards in Forschung und Experiment zu gewährleisten.