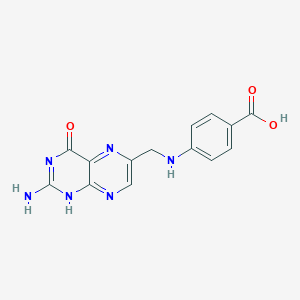
Pteroic acid
Übersicht
Beschreibung
Pteroic acid is a chemical compound related to folic acid and is an important intermediate in the synthesis of folic acid derivatives. It is a key component in the metabolic pathways of microorganisms and has been studied for its biological activity, particularly in the inhibition of enzymes like dihydrofolic acid reductase and thymidylic acid synthetase . This compound can be prepared from folic acid by microbiological techniques, which involve the removal of glutamic acid from commercially available pteroylglutamic acid using specific microorganisms .
Synthesis Analysis
The synthesis of this compound and its derivatives has been explored through various methods. One approach involves the condensation of 2-amino-3-cyano-5-chloromethylpyrazine with appropriately substituted thiols to create 10-thia analogs of this compound . Another method includes the use of α-halogenocarbonyl compounds on 4,5-diaminopyrimidines, which is notable for the spontaneous oxidation of the initially formed dihydro-pteridine . Additionally, this compound has been synthesized from folic acid by microbiological techniques, which is a convenient method for obtaining this compound by removing glutamic acid using a pteroylglutamic acid-degrading pseudomonad .
Molecular Structure Analysis
The molecular structure of this compound derivatives has been characterized using various analytical techniques. For instance, the synthesis of a bis-decyl chain derivative of this compound was structurally characterized, and its photocleavage reaction was investigated . Single-crystal X-ray structure determination has been employed to study the structure of platinum complexes with this compound derivatives, providing insights into the molecular properties relevant for applications such as dye-sensitized solar cells .
Chemical Reactions Analysis
This compound and its derivatives participate in a range of chemical reactions. For example, the photocleavage of this compound has been demonstrated in the presence of water, with no reaction observed in methanol solution . The chemical oxidation of dihydrothis compound to this compound has been used to evaluate the activity of the enzyme 7,8-dihydropteroate synthase . The regioselectivity and biological activity of substituted this compound compounds have also been explored, with several compounds showing high activity against methotrexate-sensitive and resistant microorganisms .
Physical and Chemical Properties Analysis
The physical and chemical properties of this compound and its analogs have been studied through various techniques. High-performance thin-layer chromatography (HPTLC) has been developed to determine this compound, allowing the separation of enzyme substrates and competitively formed drug analogs from the oxidized enzymatic product . Electrochemical characterization of platinum complexes with this compound derivatives has been conducted, revealing the effects of substituent position on the electrochemistry of the complexes . The lipophilicity of certain this compound derivatives, such as the bis-decyl this compound, confers hydrophobic properties that enable interaction with biomembranes .
Wissenschaftliche Forschungsanwendungen
Pteroic acid has a wide range of scientific research applications in chemistry, biology, medicine, and industry. In chemistry, it is used as a starting material to synthesize labeled folate binding protein ligands, which are employed in homogeneous chemiluminescent assay formats for the detection of folic acid . In biology, this compound is studied for its role in the biosynthesis of folates and its potential as a therapeutic agent . In medicine, this compound conjugates are being investigated for their antitumor activity and ability to mediate the selective delivery of ligands to tumors overexpressing the folate receptor . In industry, this compound is used in the production of various pharmaceuticals and diagnostic reagents .
Safety and Hazards
Zukünftige Richtungen
Wirkmechanismus
The mechanism of action of pteroic acid involves its interaction with specific molecular targets and pathways. This compound acts as an inhibitor of dihydropteroate synthase, an enzyme involved in the biosynthesis of folates . By inhibiting this enzyme, this compound disrupts the production of folates, which are essential for DNA synthesis and cell division . This mechanism of action underlies its potential therapeutic applications, particularly in the treatment of cancer and bacterial infections .
Biochemische Analyse
Biochemical Properties
In biochemical reactions, Pteroic acid interacts with various enzymes, proteins, and other biomolecules. The nature of these interactions is complex and multifaceted, involving both covalent and non-covalent bonds .
Cellular Effects
This compound: has significant effects on various types of cells and cellular processes. It influences cell function, including impacts on cell signaling pathways, gene expression, and cellular metabolism .
Molecular Mechanism
The mechanism of action of This compound is intricate. It exerts its effects at the molecular level, including binding interactions with biomolecules, enzyme inhibition or activation, and changes in gene expression .
Temporal Effects in Laboratory Settings
In laboratory settings, the effects of This compound change over time. This includes information on the product’s stability, degradation, and any long-term effects on cellular function observed in in vitro or in vivo studies .
Dosage Effects in Animal Models
The effects of This compound vary with different dosages in animal models. This includes any threshold effects observed in these studies, as well as any toxic or adverse effects at high doses .
Metabolic Pathways
This compound: is involved in several metabolic pathways, interacting with various enzymes or cofactors. This also includes any effects on metabolic flux or metabolite levels .
Transport and Distribution
This compound: is transported and distributed within cells and tissues. This includes any transporters or binding proteins that it interacts with, as well as any effects on its localization or accumulation .
Subcellular Localization
The subcellular localization of This compound and any effects on its activity or function are complex. This includes any targeting signals or post-translational modifications that direct it to specific compartments or organelles .
Vorbereitungsmethoden
Synthetic Routes and Reaction Conditions: Pteroic acid can be synthesized through various methods. One common synthetic route involves the chemical conversion of folic acid (pteroylglutamic acid) to this compound . This process typically involves the hydrolysis of folic acid under acidic conditions to remove the glutamic acid moiety, resulting in the formation of this compound .
Industrial Production Methods: Industrial production of this compound often involves the use of high-performance liquid chromatography (HPLC) for purification . The mobile phase for HPLC typically contains acetonitrile, water, and phosphoric acid, which can be replaced with formic acid for mass spectrometry-compatible applications .
Analyse Chemischer Reaktionen
Types of Reactions: Pteroic acid undergoes various chemical reactions, including oxidation, reduction, and substitution reactions . These reactions are influenced by the presence of the pteridine ring system, which contains four nitrogen atoms and exhibits a wide range of tautomerism in water .
Common Reagents and Conditions: Common reagents used in the reactions of this compound include oxidizing agents, reducing agents, and nucleophiles . For example, the oxidation of this compound can be achieved using hydrogen peroxide, while reduction can be carried out using sodium borohydride .
Major Products Formed: The major products formed from the reactions of this compound depend on the specific reaction conditions and reagents used. For instance, oxidation of this compound can yield pterin-6-carboxylic acid, while reduction can produce tetrahydrothis compound .
Vergleich Mit ähnlichen Verbindungen
Pteroic acid is structurally related to other pterin derivatives, such as pterin, neopterin, and tetrahydrobiopterin . These compounds share a common pteridine ring system but differ in their substituents and oxidation states . For example, pterin is the oxidized form of the pteridine ring system, while tetrahydrobiopterin is the fully reduced form . This compound is unique in its ability to inhibit dihydropteroate synthase and its role as an intermediate in the biosynthesis of folates .
List of Similar Compounds:- Pterin
- Neopterin
- Tetrahydrobiopterin
- Pteroylglutamic acid (Folic acid)
- Pterin-6-carboxylic acid
This compound’s unique properties and diverse applications make it a valuable compound in scientific research and industrial applications.
Eigenschaften
IUPAC Name |
4-[(2-amino-4-oxo-3H-pteridin-6-yl)methylamino]benzoic acid | |
---|---|---|
Source | PubChem | |
URL | https://pubchem.ncbi.nlm.nih.gov | |
Description | Data deposited in or computed by PubChem | |
InChI |
InChI=1S/C14H12N6O3/c15-14-19-11-10(12(21)20-14)18-9(6-17-11)5-16-8-3-1-7(2-4-8)13(22)23/h1-4,6,16H,5H2,(H,22,23)(H3,15,17,19,20,21) | |
Source | PubChem | |
URL | https://pubchem.ncbi.nlm.nih.gov | |
Description | Data deposited in or computed by PubChem | |
InChI Key |
JOAQINSXLLMRCV-UHFFFAOYSA-N | |
Source | PubChem | |
URL | https://pubchem.ncbi.nlm.nih.gov | |
Description | Data deposited in or computed by PubChem | |
Canonical SMILES |
C1=CC(=CC=C1C(=O)O)NCC2=CN=C3C(=N2)C(=O)NC(=N3)N | |
Source | PubChem | |
URL | https://pubchem.ncbi.nlm.nih.gov | |
Description | Data deposited in or computed by PubChem | |
Molecular Formula |
C14H12N6O3 | |
Source | PubChem | |
URL | https://pubchem.ncbi.nlm.nih.gov | |
Description | Data deposited in or computed by PubChem | |
DSSTOX Substance ID |
DTXSID40152279 | |
Record name | Pteroic acid | |
Source | EPA DSSTox | |
URL | https://comptox.epa.gov/dashboard/DTXSID40152279 | |
Description | DSSTox provides a high quality public chemistry resource for supporting improved predictive toxicology. | |
Molecular Weight |
312.28 g/mol | |
Source | PubChem | |
URL | https://pubchem.ncbi.nlm.nih.gov | |
Description | Data deposited in or computed by PubChem | |
CAS RN |
119-24-4 | |
Record name | Pteroic acid | |
Source | CAS Common Chemistry | |
URL | https://commonchemistry.cas.org/detail?cas_rn=119-24-4 | |
Description | CAS Common Chemistry is an open community resource for accessing chemical information. Nearly 500,000 chemical substances from CAS REGISTRY cover areas of community interest, including common and frequently regulated chemicals, and those relevant to high school and undergraduate chemistry classes. This chemical information, curated by our expert scientists, is provided in alignment with our mission as a division of the American Chemical Society. | |
Explanation | The data from CAS Common Chemistry is provided under a CC-BY-NC 4.0 license, unless otherwise stated. | |
Record name | Pteroic acid | |
Source | ChemIDplus | |
URL | https://pubchem.ncbi.nlm.nih.gov/substance/?source=chemidplus&sourceid=0000119244 | |
Description | ChemIDplus is a free, web search system that provides access to the structure and nomenclature authority files used for the identification of chemical substances cited in National Library of Medicine (NLM) databases, including the TOXNET system. | |
Record name | Pteroic acid | |
Source | DrugBank | |
URL | https://www.drugbank.ca/drugs/DB04196 | |
Description | The DrugBank database is a unique bioinformatics and cheminformatics resource that combines detailed drug (i.e. chemical, pharmacological and pharmaceutical) data with comprehensive drug target (i.e. sequence, structure, and pathway) information. | |
Explanation | Creative Common's Attribution-NonCommercial 4.0 International License (http://creativecommons.org/licenses/by-nc/4.0/legalcode) | |
Record name | Pteroic acid | |
Source | DTP/NCI | |
URL | https://dtp.cancer.gov/dtpstandard/servlet/dwindex?searchtype=NSC&outputformat=html&searchlist=14972 | |
Description | The NCI Development Therapeutics Program (DTP) provides services and resources to the academic and private-sector research communities worldwide to facilitate the discovery and development of new cancer therapeutic agents. | |
Explanation | Unless otherwise indicated, all text within NCI products is free of copyright and may be reused without our permission. Credit the National Cancer Institute as the source. | |
Record name | Pteroic acid | |
Source | EPA DSSTox | |
URL | https://comptox.epa.gov/dashboard/DTXSID40152279 | |
Description | DSSTox provides a high quality public chemistry resource for supporting improved predictive toxicology. | |
Record name | PTEROIC ACID | |
Source | FDA Global Substance Registration System (GSRS) | |
URL | https://gsrs.ncats.nih.gov/ginas/app/beta/substances/8258W48TBZ | |
Description | The FDA Global Substance Registration System (GSRS) enables the efficient and accurate exchange of information on what substances are in regulated products. Instead of relying on names, which vary across regulatory domains, countries, and regions, the GSRS knowledge base makes it possible for substances to be defined by standardized, scientific descriptions. | |
Explanation | Unless otherwise noted, the contents of the FDA website (www.fda.gov), both text and graphics, are not copyrighted. They are in the public domain and may be republished, reprinted and otherwise used freely by anyone without the need to obtain permission from FDA. Credit to the U.S. Food and Drug Administration as the source is appreciated but not required. | |
Retrosynthesis Analysis
AI-Powered Synthesis Planning: Our tool employs the Template_relevance Pistachio, Template_relevance Bkms_metabolic, Template_relevance Pistachio_ringbreaker, Template_relevance Reaxys, Template_relevance Reaxys_biocatalysis model, leveraging a vast database of chemical reactions to predict feasible synthetic routes.
One-Step Synthesis Focus: Specifically designed for one-step synthesis, it provides concise and direct routes for your target compounds, streamlining the synthesis process.
Accurate Predictions: Utilizing the extensive PISTACHIO, BKMS_METABOLIC, PISTACHIO_RINGBREAKER, REAXYS, REAXYS_BIOCATALYSIS database, our tool offers high-accuracy predictions, reflecting the latest in chemical research and data.
Strategy Settings
Precursor scoring | Relevance Heuristic |
---|---|
Min. plausibility | 0.01 |
Model | Template_relevance |
Template Set | Pistachio/Bkms_metabolic/Pistachio_ringbreaker/Reaxys/Reaxys_biocatalysis |
Top-N result to add to graph | 6 |
Feasible Synthetic Routes
Haftungsausschluss und Informationen zu In-Vitro-Forschungsprodukten
Bitte beachten Sie, dass alle Artikel und Produktinformationen, die auf BenchChem präsentiert werden, ausschließlich zu Informationszwecken bestimmt sind. Die auf BenchChem zum Kauf angebotenen Produkte sind speziell für In-vitro-Studien konzipiert, die außerhalb lebender Organismen durchgeführt werden. In-vitro-Studien, abgeleitet von dem lateinischen Begriff "in Glas", beinhalten Experimente, die in kontrollierten Laborumgebungen unter Verwendung von Zellen oder Geweben durchgeführt werden. Es ist wichtig zu beachten, dass diese Produkte nicht als Arzneimittel oder Medikamente eingestuft sind und keine Zulassung der FDA für die Vorbeugung, Behandlung oder Heilung von medizinischen Zuständen, Beschwerden oder Krankheiten erhalten haben. Wir müssen betonen, dass jede Form der körperlichen Einführung dieser Produkte in Menschen oder Tiere gesetzlich strikt untersagt ist. Es ist unerlässlich, sich an diese Richtlinien zu halten, um die Einhaltung rechtlicher und ethischer Standards in Forschung und Experiment zu gewährleisten.