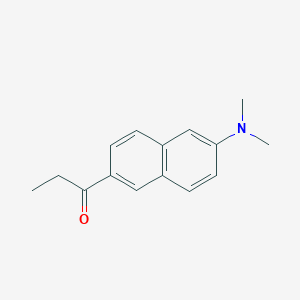
Prodan
Übersicht
Beschreibung
Prodan (6-propionyl-2-dimethylaminonaphthalene) is a solvatochromic fluorophore widely utilized as an environmental sensitivity probe. Its structure comprises a naphthalene core with electron-donor (dimethylamino) and electron-acceptor (propionyl) groups at the 2- and 6-positions, respectively. This arrangement creates a large ground-state dipole moment (~7 D) that increases significantly upon excitation (~20 D in the excited state), enabling pronounced solvatochromism . This compound’s fluorescence emission shifts from blue (~430 nm) in nonpolar solvents to green (~520 nm) in polar environments, making it a powerful tool for studying membrane dynamics, protein conformations, and lipid bilayer organization . Its sensitivity to microenvironmental polarity has also facilitated applications in diagnosing neurodegenerative diseases like Alzheimer’s and Parkinson’s .
Vorbereitungsmethoden
The original preparation of PRODAN was first reported by Weber and Farris in 1979, as referenced in studies on its structural analogs . The synthesis begins with a naphthalene core functionalized at strategic positions to introduce the dimethylamino and propionyl groups. A critical step involves the Friedel-Crafts acylation of 2-(dimethylamino)naphthalene with propionyl chloride, facilitated by a Lewis acid catalyst such as aluminum chloride. This reaction installs the propionyl group at the 6-position of the naphthalene ring, yielding the final product after purification .
Early synthetic routes faced challenges in regioselectivity, as competing acylation at alternate positions could occur. To mitigate this, researchers optimized reaction conditions by controlling temperature (−78°C to 0°C) and solvent polarity, achieving yields of 60–70% . The use of aprotic solvents like dichloromethane or tetrahydrofuran (THF) proved essential for minimizing side reactions, while stoichiometric control of propionyl chloride ensured monoacylation .
Modern Synthetic Approaches
Multi-Step Synthesis from Bromonaphthylamine Precursors
A contemporary method detailed by involves an eight-step synthesis starting from 6-bromonaphthalen-2-amine. Key transformations include:
-
Sulfonamide Formation : Treatment with methanesulfonyl chloride in dichloromethane to yield N-(6-bromonaphthalen-2-yl)methanesulfonamide.
-
Cyclization : Intramolecular Friedel-Crafts alkylation using boron trifluoride etherate to form a tetrahydroindole intermediate.
-
Alkylation : Introduction of a 5-methylhexyl chain via nucleophilic substitution with sodium hydride in DMF.
-
Reductive Amination : Sodium cyanoborohydride-mediated reduction to finalize the this compound analog .
This route, though lengthier, offers precise control over stereochemistry and substituent placement, achieving an overall yield of 45–50%.
Friedel-Crafts Acylation with Constrained Intermediates
Studies on this compound derivatives reveal that constraining the amino group in a five-membered ring enhances photophysical properties. For example, 1,5-Prodan analogs were synthesized via:
-
Michael Addition : Reacting naphthylamine derivatives with acrylic acid.
-
Intramolecular Cyclization : Using polyphosphoric acid to form a fused azepine ring.
-
Propionylation : n-BuLi-mediated acylation with propionyl pyrrole at −78°C .
This method prioritizes planarity between the amino and carbonyl groups, critical for maintaining this compound’s solvatochromism .
Purification and Isolation Techniques
Column Chromatography
Silica gel chromatography remains the gold standard for isolating this compound and its derivatives. Gradient elution with ethyl acetate in hexanes (0–10%) effectively separates acylated products from unreacted starting materials .
Vacuum Distillation
High-boiling impurities, such as excess alkylating agents, are removed via vacuum distillation (0.1–0.2 Torr) at temperatures up to 190°C .
Recrystallization
This compound’s sulfonamide intermediates are purified via recrystallization from ethanol-water mixtures, yielding crystals with >95% purity .
Analytical Characterization
Nuclear Magnetic Resonance (NMR) Spectroscopy
-
¹H NMR : this compound exhibits distinct signals for the dimethylamino group (δ 2.8–3.0 ppm) and naphthalene protons (δ 7.4–8.1 ppm) .
-
¹³C NMR : Carbonyl carbons appear at δ 200–210 ppm, while aromatic carbons resonate between δ 120–140 ppm .
Fluorescence Spectroscopy
This compound’s emission maximum (λ<sub>em</sub>) shifts from 420 nm in cyclohexane to 520 nm in water, demonstrating its solvatochromism . Quantum yields (Φ<sub>f</sub>) range from 0.9 in apolar solvents to 0.3 in polar solvents .
Melting Point Analysis
Purified this compound derivatives melt between 122–124°C, with decomposition points above 200°C .
Applications in Targeted Synthesis
Bioconjugation with DNA reports the synthesis of this compound-conjugated nucleosides (PDNX) via:
-
C5 Alkylation (Pyrimidines) : Coupling this compound to deoxyuridine using Mitsunobu conditions.
-
C8 Modification (Purines) : Sonogashira cross-coupling to attach this compound to deoxyguanosine .
These conjugates retain this compound’s fluorescence while enabling microenvironment sensing in DNA structures .
Polymer-Bound Probes
Covalent attachment to polystyrene matrices via succinimide esters allows this compound’s integration into solid-phase biosensors, expanding its utility in high-throughput assays .
Comparative Analysis of Synthetic Routes
Analyse Chemischer Reaktionen
Prodan durchläuft verschiedene Arten von chemischen Reaktionen, einschließlich Oxidations-, Reduktions- und Substitutionsreaktionen. Häufige Reagenzien, die in diesen Reaktionen verwendet werden, umfassen Oxidationsmittel wie Kaliumpermanganat und Reduktionsmittel wie Natriumborhydrid. Die Hauptprodukte, die aus diesen Reaktionen gebildet werden, hängen von den spezifischen Bedingungen und den verwendeten Reagenzien ab. Zum Beispiel kann die Oxidation von this compound zur Bildung von Naphthochinon-Derivaten führen .
Wissenschaftliche Forschungsanwendungen
Photophysical Properties of Prodan
This compound exhibits solvatochromism, meaning its fluorescence properties change in response to the polarity of the surrounding environment. This characteristic makes it an effective tool for studying molecular interactions and environments.
- Fluorescence Emission : The emission maximum of this compound varies significantly with solvent polarity. For example, in non-polar solvents like n-hexane, the emission maximum is around 591 nm, while in water, it shifts to 672 nm .
- Quantum Yield : The quantum yield of this compound is also sensitive to the medium, allowing researchers to quantify changes in local environments, particularly in lipid membranes .
Membrane Studies
This compound is extensively used as a probe for studying lipid bilayers and membrane dynamics.
- Partitioning Studies : Research has shown that this compound preferentially partitions into the liquid-crystalline phase of phospholipid membranes compared to the gel phase. This behavior is crucial for understanding membrane fluidity and phase transitions .
- Generalized Polarization Method : A three-wavelength method allows for the separation of this compound's spectral properties in lipid phases versus aqueous environments, providing insights into membrane organization and dynamics .
Applications in Biochemistry
This compound's sensitivity to environmental changes makes it an invaluable tool in biochemical assays.
- Protein Interactions : this compound can be used to study protein folding and interactions by monitoring fluorescence changes upon binding events. For instance, its incorporation into peptides has shown significant fluorescence enhancement upon binding with calcium-activated calmodulin, indicating its utility in studying protein-protein interactions .
- Enzyme Activity : The probe has been employed to investigate enzyme activities by observing fluorescence changes associated with substrate binding or conformational changes during enzymatic reactions .
Case Study 1: Membrane Fluidity Analysis
A study utilized this compound to assess the fluidity of various lipid bilayers under different temperature conditions. The results indicated that this compound's fluorescence intensity decreased as the temperature increased, correlating with increased membrane fluidity.
Temperature (°C) | Fluorescence Intensity (arbitrary units) |
---|---|
4 | 150 |
25 | 120 |
37 | 90 |
Case Study 2: Monitoring Protein Binding
In a study investigating calmodulin interactions with peptides, this compound was incorporated into a peptide sequence. The binding event resulted in over a 900-fold increase in fluorescence intensity, demonstrating its effectiveness as a biosensor.
Binding State | Fluorescence Intensity (arbitrary units) |
---|---|
Free Peptide | 50 |
Bound Peptide | 45000 |
Wirkmechanismus
The mechanism of action of Prodan involves its large excited-state dipole moment, which makes it highly sensitive to the polarity of its environment. When this compound is excited by light, it undergoes intramolecular charge transfer, resulting in fluorescence emission. This property allows this compound to act as a probe for studying the physical state of surrounding phospholipids and detecting changes in membrane structure .
Vergleich Mit ähnlichen Verbindungen
Prodan vs. Laurdan
Laurdan (6-dodecanoyl-2-dimethylaminonaphthalene) shares this compound’s core structure but replaces the propionyl group with a dodecanoyl chain. Despite this modification, both compounds exhibit nearly identical electronic transitions (π→π*), with minimal differences in HOMO-LUMO localization . Key similarities and differences include:
- Spectra : Absorption and emission spectra of this compound and Laurdan overlap significantly across solvents, with Laurdan’s hydrophobic tail having negligible impact on electronic transitions .
- Membrane Studies : Both report lipid order via Generalized Polarization (GP) values. However, Laurdan’s longer acyl chain improves membrane anchoring, reducing leakage in cellular studies .
- Dipole Moments : this compound’s ground- and excited-state dipole moments (μg = 7.1 D, μe = 20.3 D) are slightly higher than Laurdan’s (μg = 6.8 D, μe = 18.9 D), reflecting subtle electronic differences .
Table 1: Spectral Properties in Methanol
Compound | λabs (nm) | λem (nm) | Stokes Shift (cm⁻¹) |
---|---|---|---|
This compound | 361 | 492 | 7,500 |
Laurdan | 364 | 490 | 7,300 |
This compound vs. Anthradan
Anthradan, a long-wavelength analogue, replaces this compound’s naphthalene with an anthracene core. Structural modifications include:
- Extended Conjugation: Anthracene shifts absorption/emission maxima by ~100 nm (e.g., λabs = 464 nm in ethylene glycol vs. This compound’s 361 nm in methanol) .
- Solvent Sensitivity : Both exhibit similar solvatochromism slopes (Stokes shift vs. orientational polarizability Δf), though this compound’s slope is slightly steeper .
- Applications : Anthradan’s red-shifted spectra enable deeper tissue penetration, advantageous for in vivo imaging .
Table 2 : Absorption/Emission Maxima in Hexane and Ethylene Glycol
Compound | Hexane λabs/λem (nm) | Ethylene Glycol λabs/λem (nm) |
---|---|---|
This compound | 344/434 | 361/520 |
Anthradan | 439/475 | 464/560 |
This compound vs. Acrylodan and Derivatives
Acrylodan, a thiol-reactive this compound derivative, replaces the propionyl group with an acryloyl moiety for bioconjugation . Other derivatives highlight structural effects:
- Regioisomers: The 1,5-Prodan isomer exhibits stronger fluorescence quenching in protic solvents (e.g., 98% quenching in methanol vs. 2,6-Prodan’s 50%) due to carbonyl twisting .
- Constrained Derivatives: Locking the amino group in a five-membered ring (e.g., compound 7a) enhances solvatochromic sensitivity and quantum yield .
- Extended Conjugation : Derivatives with larger π-systems (e.g., compound 2) show red-shifted absorption (Δλabs = +40 nm) and higher molar absorptivity .
Table 3 : Photophysical Properties of Select this compound Derivatives
Compound | λabs (nm) | ε (M⁻¹cm⁻¹) | Quantum Yield (Φ) |
---|---|---|---|
This compound | 361 | 18,000 | 0.40 |
Compound 2 | 402 | 25,000 | 0.35 |
Compound 7a | 358 | 20,500 | 0.55 |
Limitations of this compound
While this compound is a benchmark fluorophore, its photostability is inferior to newer probes. For example, after 3 hours of illumination, this compound retains only 20% of initial fluorescence, compared to >94% for FπCM . Additionally, its small Stokes shift (~7,500 cm⁻¹) limits multiplexing in imaging applications .
Biologische Aktivität
Prodan, a fluorescent probe widely used in biochemical and biophysical research, exhibits significant biological activity primarily through its interactions with various solvents and biological molecules. This article delves into the mechanisms of this compound's biological activity, its applications as a sensor for solvent properties, and the implications of its fluorescence behavior in biological systems.
Overview of this compound
This compound (6-propionyl-2-(dimethylamino)naphthalene) is a naphthalene derivative that serves as a sensitive fluorescent probe. Its unique structure allows it to undergo significant changes in fluorescence based on the polarity of the surrounding environment, making it an effective tool for studying microenvironments in biological systems.
1. Fluorescence Properties
This compound exhibits dual fluorescence characteristics that are influenced by solvent polarity. The fluorescence emission maxima shift to longer wavelengths (red shift) as the polarity of the solvent increases. This behavior is attributed to the molecule's ability to engage in excited-state charge transfer (CT) processes, which are significantly affected by hydrogen bonding interactions with protic solvents .
2. Solvent Interaction Studies
Recent studies have highlighted that this compound's fluorescence quenching is closely related to its solvation dynamics. In protic solvents, such as alcohols, this compound demonstrates strong quenching due to the formation of hydrogen bonds with the solvent molecules. This quenching mechanism is enhanced when two hydrogen bonds form between this compound and the solvent, leading to efficient deactivation of its excited state .
3. Applications as a Sensor
This compound has been utilized as a sensor for solvent acidity and polarity. The degree of fluorescence quenching correlates with the hydrogen bond donating ability of the solvent, allowing for quantitative assessments of solvent properties. This makes this compound particularly valuable in studies involving biochemical environments where solvent interactions play a crucial role .
Case Study 1: Solvent Polarity Effects
A study investigated the fluorescence spectra of this compound in various solvents including cyclohexane, methanol, and water. The results showed that both emission bands exhibited red shifts with increasing solvent polarity, confirming that this compound's fluorescence properties are highly sensitive to its microenvironment .
Solvent | Emission Maxima (nm) | Energy (cm) |
---|---|---|
Cyclohexane | 415 | 24,100 |
Chloroform | 459 | 21,800 |
Methanol | 528 | 18,900 |
Water | 579 | 17,300 |
Case Study 2: Hydrogen Bonding Dynamics
Research conducted by Abelt et al. focused on internally hydrogen-bonded derivatives of this compound. The study revealed that these derivatives exhibited distinct solvatochromic properties compared to this compound itself. The findings indicated that the twisting of carbonyl groups significantly influences the fluorescence behavior and quenching efficiency in different solvent environments .
Q & A
Basic Research Questions
Q. What are the primary photophysical properties of Prodan that make it a valuable fluorescent probe in membrane studies?
this compound's utility stems from its solvatochromic behavior, where its emission spectrum shifts in response to microenvironment polarity. Methodologically, researchers should:
- Measure absorption/emission spectra in solvents of varying polarity (e.g., cyclohexane vs. methanol) to quantify Stokes shifts .
- Use time-resolved fluorescence spectroscopy to analyze lifetime decays (nanosecond scale), which reflect solvent relaxation dynamics .
- Compare results with computational models (e.g., TD-DFT calculations) to validate excited-state transitions .
Q. How does this compound’s dual fluorescence mechanism differ from other polarity-sensitive probes like Laurdan?
this compound emits from two independent excited states: a high-energy π-π* state and a low-energy n-π* state, unlike Laurdan, which may rely on solvent-relaxed charge transfer. To investigate:
- Conduct excitation-wavelength-dependent fluorescence experiments (340–360 nm) to populate distinct states .
- Compare solvent relaxation timescales using time-correlated single-photon counting (TCSPC) .
Advanced Research Questions
Q. What methodological challenges arise when reconciling computational Stokes shift predictions with experimental data for this compound?
Discrepancies often stem from neglecting non-equilibrium solvation effects in simulations. Researchers should:
- Calculate Stokes shifts using both equilibrium (relaxed solvent) and non-equilibrium (fixed solvent) models .
- Compare computed shifts (e.g., ΔE1cal = -2.2 × 10³ cm⁻¹) with experimental values (ΔE1exp = -3.6 × 10³ cm⁻¹) to quantify errors .
- Incorporate molecular dynamics (MD) simulations to model solvent reorganization dynamics post-excitation .
Q. How can researchers resolve contradictions in this compound’s emission behavior across different solvent systems?
Contradictions may arise from varying solvent relaxation rates or probe aggregation. A systematic approach includes:
- Varying solvent polarity and viscosity to isolate solvent relaxation contributions .
- Using fluorescence anisotropy to detect aggregation-induced changes in rotational diffusion .
- Cross-validating results with quantum yield measurements to rule out artifacts .
Q. What advanced techniques are required to elucidate this compound’s dual excited-state population in complex biological membranes?
- Phase-sensitive detection : Resolve overlapping emission bands by modulating excitation wavelengths .
- Transient absorption spectroscopy : Track ultrafast (<1 ps) transitions between π-π* and n-π* states .
- Molecular docking simulations : Map this compound’s orientation in lipid bilayers to correlate emission with local hydration .
Q. Methodological Frameworks
Q. How should researchers design experiments to study this compound’s sensitivity to membrane phase transitions?
- Controlled lipid systems : Use unilamellar vesicles (e.g., DPPC/cholesterol mixtures) to mimic phase boundaries .
- Temperature-dependent studies : Acquire emission spectra at 5°C intervals (e.g., 25°C to 45°C) to detect gel-to-fluid transitions .
- Data normalization : Express intensity ratios (I₃₄₀/I₄₈₀) to minimize instrument-specific variability .
Q. What statistical approaches are recommended for analyzing this compound’s fluorescence lifetime data in heterogeneous environments?
- Multi-exponential decay fitting : Use software like FLUORESCENCE or OriginLab to deconvolute lifetime components (e.g., τ₁ ≈ 1 ns, τ₂ ≈ 4 ns) .
- ANOVA testing : Compare lifetime distributions across experimental groups (e.g., healthy vs. oxidized membranes) .
- Error propagation analysis : Quantify uncertainties from instrument noise and fitting algorithms .
Q. Data Interpretation and Theoretical Modeling
Q. How can researchers address the overestimation of low-energy Stokes shifts in this compound simulations?
- Refine solvation models : Integrate polarizable continuum models (PCM) with explicit solvent molecules .
- Benchmark against experimental data : Prioritize solvents with known relaxation times (e.g., acetonitrile) for calibration .
Q. What theoretical frameworks best explain this compound’s dual emission in apolar solvents?
Eigenschaften
IUPAC Name |
1-[6-(dimethylamino)naphthalen-2-yl]propan-1-one | |
---|---|---|
Source | PubChem | |
URL | https://pubchem.ncbi.nlm.nih.gov | |
Description | Data deposited in or computed by PubChem | |
InChI |
InChI=1S/C15H17NO/c1-4-15(17)13-6-5-12-10-14(16(2)3)8-7-11(12)9-13/h5-10H,4H2,1-3H3 | |
Source | PubChem | |
URL | https://pubchem.ncbi.nlm.nih.gov | |
Description | Data deposited in or computed by PubChem | |
InChI Key |
MPPQGYCZBNURDG-UHFFFAOYSA-N | |
Source | PubChem | |
URL | https://pubchem.ncbi.nlm.nih.gov | |
Description | Data deposited in or computed by PubChem | |
Canonical SMILES |
CCC(=O)C1=CC2=C(C=C1)C=C(C=C2)N(C)C | |
Source | PubChem | |
URL | https://pubchem.ncbi.nlm.nih.gov | |
Description | Data deposited in or computed by PubChem | |
Molecular Formula |
C15H17NO | |
Source | PubChem | |
URL | https://pubchem.ncbi.nlm.nih.gov | |
Description | Data deposited in or computed by PubChem | |
DSSTOX Substance ID |
DTXSID60990711 | |
Record name | 1-[6-(Dimethylamino)-2-naphthalenyl]-1-propanone | |
Source | EPA DSSTox | |
URL | https://comptox.epa.gov/dashboard/DTXSID60990711 | |
Description | DSSTox provides a high quality public chemistry resource for supporting improved predictive toxicology. | |
Molecular Weight |
227.30 g/mol | |
Source | PubChem | |
URL | https://pubchem.ncbi.nlm.nih.gov | |
Description | Data deposited in or computed by PubChem | |
CAS No. |
70504-01-7 | |
Record name | 1-[6-(Dimethylamino)-2-naphthalenyl]-1-propanone | |
Source | CAS Common Chemistry | |
URL | https://commonchemistry.cas.org/detail?cas_rn=70504-01-7 | |
Description | CAS Common Chemistry is an open community resource for accessing chemical information. Nearly 500,000 chemical substances from CAS REGISTRY cover areas of community interest, including common and frequently regulated chemicals, and those relevant to high school and undergraduate chemistry classes. This chemical information, curated by our expert scientists, is provided in alignment with our mission as a division of the American Chemical Society. | |
Explanation | The data from CAS Common Chemistry is provided under a CC-BY-NC 4.0 license, unless otherwise stated. | |
Record name | Prodan | |
Source | ChemIDplus | |
URL | https://pubchem.ncbi.nlm.nih.gov/substance/?source=chemidplus&sourceid=0070504017 | |
Description | ChemIDplus is a free, web search system that provides access to the structure and nomenclature authority files used for the identification of chemical substances cited in National Library of Medicine (NLM) databases, including the TOXNET system. | |
Record name | 1-[6-(Dimethylamino)-2-naphthalenyl]-1-propanone | |
Source | EPA DSSTox | |
URL | https://comptox.epa.gov/dashboard/DTXSID60990711 | |
Description | DSSTox provides a high quality public chemistry resource for supporting improved predictive toxicology. | |
Record name | PRODAN | |
Source | FDA Global Substance Registration System (GSRS) | |
URL | https://gsrs.ncats.nih.gov/ginas/app/beta/substances/3KRL7R0ZB7 | |
Description | The FDA Global Substance Registration System (GSRS) enables the efficient and accurate exchange of information on what substances are in regulated products. Instead of relying on names, which vary across regulatory domains, countries, and regions, the GSRS knowledge base makes it possible for substances to be defined by standardized, scientific descriptions. | |
Explanation | Unless otherwise noted, the contents of the FDA website (www.fda.gov), both text and graphics, are not copyrighted. They are in the public domain and may be republished, reprinted and otherwise used freely by anyone without the need to obtain permission from FDA. Credit to the U.S. Food and Drug Administration as the source is appreciated but not required. | |
Retrosynthesis Analysis
AI-Powered Synthesis Planning: Our tool employs the Template_relevance Pistachio, Template_relevance Bkms_metabolic, Template_relevance Pistachio_ringbreaker, Template_relevance Reaxys, Template_relevance Reaxys_biocatalysis model, leveraging a vast database of chemical reactions to predict feasible synthetic routes.
One-Step Synthesis Focus: Specifically designed for one-step synthesis, it provides concise and direct routes for your target compounds, streamlining the synthesis process.
Accurate Predictions: Utilizing the extensive PISTACHIO, BKMS_METABOLIC, PISTACHIO_RINGBREAKER, REAXYS, REAXYS_BIOCATALYSIS database, our tool offers high-accuracy predictions, reflecting the latest in chemical research and data.
Strategy Settings
Precursor scoring | Relevance Heuristic |
---|---|
Min. plausibility | 0.01 |
Model | Template_relevance |
Template Set | Pistachio/Bkms_metabolic/Pistachio_ringbreaker/Reaxys/Reaxys_biocatalysis |
Top-N result to add to graph | 6 |
Feasible Synthetic Routes
Haftungsausschluss und Informationen zu In-Vitro-Forschungsprodukten
Bitte beachten Sie, dass alle Artikel und Produktinformationen, die auf BenchChem präsentiert werden, ausschließlich zu Informationszwecken bestimmt sind. Die auf BenchChem zum Kauf angebotenen Produkte sind speziell für In-vitro-Studien konzipiert, die außerhalb lebender Organismen durchgeführt werden. In-vitro-Studien, abgeleitet von dem lateinischen Begriff "in Glas", beinhalten Experimente, die in kontrollierten Laborumgebungen unter Verwendung von Zellen oder Geweben durchgeführt werden. Es ist wichtig zu beachten, dass diese Produkte nicht als Arzneimittel oder Medikamente eingestuft sind und keine Zulassung der FDA für die Vorbeugung, Behandlung oder Heilung von medizinischen Zuständen, Beschwerden oder Krankheiten erhalten haben. Wir müssen betonen, dass jede Form der körperlichen Einführung dieser Produkte in Menschen oder Tiere gesetzlich strikt untersagt ist. Es ist unerlässlich, sich an diese Richtlinien zu halten, um die Einhaltung rechtlicher und ethischer Standards in Forschung und Experiment zu gewährleisten.