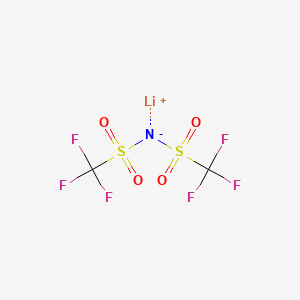
Lithium-Bis(trifluormethylsulfonyl)imid
Übersicht
Beschreibung
Bis(trifluoromethane)sulfonimide lithium salt is a synthetic reagent.
Wissenschaftliche Forschungsanwendungen
Organische Solarzellen
Li-TFSI kann als chemische Additive verwendet werden, um die Stromwandlungseffizienz in Porphyrin-basierten organischen Solarzellen zu verbessern .
Polymerisierte ionische Flüssigkeiten
Es dient als Reagenz bei der Herstellung von Imidazolium-Kern-tragenden monomeren ionischen Flüssigkeiten, die dann zur Entwicklung von polymerisierten ionischen Flüssigkeiten verwendet werden .
Organische Elektrolyt-basierte Lithiumbatterien
Li-TFSI wird bei der Herstellung von organischen Elektrolyt-basierten Lithiumbatterien eingesetzt und trägt zur Entwicklung von Hochleistungs-Energiespeichern bei .
Chirale Imidazoliumsalze
Die Verbindung ist an der Synthese von chiralen Imidazoliumsalzen durch einen Anionenaustauschprozess beteiligt, der Auswirkungen auf die asymmetrische Synthese und Katalyse hat .
Seltenerd-Lewis-Säure-Katalysatoren
Li-TFSI findet Anwendung bei der Herstellung von Seltenerd-Lewis-Säure-Katalysatoren, die für verschiedene chemische Reaktionen und Prozesse wichtig sind .
Flüssige Elektrolyte für Lithium-Ionen-Batterien
Es spielt eine Schlüsselrolle in kommerziellen Lithium-Ionen-Batterien als Teil des flüssigen Elektrolyten, der die Leitung von Lithiumionen zwischen Kathode und Anode ermöglicht .
Biochemische Analyse
Biochemical Properties
Lithium bis((trifluoromethyl)sulfonyl)azanide plays a significant role in biochemical reactions, particularly in the context of its interactions with enzymes, proteins, and other biomolecules. The compound’s anion, bistriflimide, coordinates weakly with cations, which can influence the activity of various enzymes and proteins. For instance, it has been shown to interact with carbonyl groups in certain covalent organic frameworks, forming hydrogen bonds that can affect the transfer number of lithium ions . These interactions can modulate the activity of enzymes involved in metabolic pathways, thereby influencing biochemical reactions.
Cellular Effects
Lithium bis((trifluoromethyl)sulfonyl)azanide has notable effects on various types of cells and cellular processes. It influences cell function by affecting cell signaling pathways, gene expression, and cellular metabolism. The compound’s high solubility and ability to coordinate weakly with cations allow it to penetrate cell membranes and interact with intracellular components. This can lead to alterations in cell signaling pathways, such as those involving calcium ions, and changes in gene expression that impact cellular metabolism .
Molecular Mechanism
The molecular mechanism of action of lithium bis((trifluoromethyl)sulfonyl)azanide involves its interactions with biomolecules at the molecular level. The compound’s anion, bistriflimide, coordinates weakly with cations, which can lead to enzyme inhibition or activation. For example, it can inhibit enzymes involved in the degradation of certain metabolites, thereby altering metabolic flux. Additionally, lithium bis((trifluoromethyl)sulfonyl)azanide can bind to specific proteins, leading to changes in their conformation and activity .
Temporal Effects in Laboratory Settings
In laboratory settings, the effects of lithium bis((trifluoromethyl)sulfonyl)azanide can change over time. The compound’s stability and degradation are critical factors that influence its long-term effects on cellular function. Studies have shown that lithium bis((trifluoromethyl)sulfonyl)azanide can cause damage to the nervous system through prolonged or repeated exposure . Additionally, its high solubility in water ensures that it remains active in aqueous environments, maintaining its biochemical effects over extended periods.
Dosage Effects in Animal Models
The effects of lithium bis((trifluoromethyl)sulfonyl)azanide vary with different dosages in animal models. At low doses, the compound can enhance the activity of certain enzymes and proteins, leading to beneficial effects on cellular function. At high doses, lithium bis((trifluoromethyl)sulfonyl)azanide can cause toxic or adverse effects, such as severe skin burns and eye damage . Threshold effects have been observed, indicating that there is a specific dosage range within which the compound exerts its optimal effects without causing toxicity.
Metabolic Pathways
Lithium bis((trifluoromethyl)sulfonyl)azanide is involved in various metabolic pathways, interacting with enzymes and cofactors that regulate metabolic flux. The compound’s anion, bistriflimide, can inhibit enzymes involved in the degradation of certain metabolites, leading to changes in metabolite levels. Additionally, lithium bis((trifluoromethyl)sulfonyl)azanide can affect the activity of enzymes that regulate the synthesis and breakdown of key biomolecules, thereby influencing overall metabolic pathways .
Transport and Distribution
Within cells and tissues, lithium bis((trifluoromethyl)sulfonyl)azanide is transported and distributed through interactions with transporters and binding proteins. The compound’s high solubility in water facilitates its movement across cell membranes and its accumulation in specific cellular compartments. This can lead to localized effects on cellular function, depending on the concentration and distribution of lithium bis((trifluoromethyl)sulfonyl)azanide within the cell .
Subcellular Localization
The subcellular localization of lithium bis((trifluoromethyl)sulfonyl)azanide is influenced by targeting signals and post-translational modifications that direct it to specific compartments or organelles. The compound can accumulate in organelles such as mitochondria and lysosomes, where it can exert its effects on cellular metabolism and function. This localization is critical for its activity, as it allows lithium bis((trifluoromethyl)sulfonyl)azanide to interact with specific biomolecules and modulate their activity .
Eigenschaften
CAS-Nummer |
90076-65-6 |
---|---|
Molekularformel |
C2HF6LiNO4S2 |
Molekulargewicht |
288.1 g/mol |
IUPAC-Name |
lithium;bis(trifluoromethylsulfonyl)azanide |
InChI |
InChI=1S/C2HF6NO4S2.Li/c3-1(4,5)14(10,11)9-15(12,13)2(6,7)8;/h9H; |
InChI-Schlüssel |
UGEOMRBXRFUYJH-UHFFFAOYSA-N |
SMILES |
[Li+].C(F)(F)(F)S(=O)(=O)[N-]S(=O)(=O)C(F)(F)F |
Kanonische SMILES |
[Li].C(F)(F)(F)S(=O)(=O)NS(=O)(=O)C(F)(F)F |
90076-65-6 | |
Physikalische Beschreibung |
PelletsLargeCrystals |
Piktogramme |
Corrosive; Acute Toxic; Health Hazard |
Herkunft des Produkts |
United States |
Synthesis routes and methods
Procedure details
Retrosynthesis Analysis
AI-Powered Synthesis Planning: Our tool employs the Template_relevance Pistachio, Template_relevance Bkms_metabolic, Template_relevance Pistachio_ringbreaker, Template_relevance Reaxys, Template_relevance Reaxys_biocatalysis model, leveraging a vast database of chemical reactions to predict feasible synthetic routes.
One-Step Synthesis Focus: Specifically designed for one-step synthesis, it provides concise and direct routes for your target compounds, streamlining the synthesis process.
Accurate Predictions: Utilizing the extensive PISTACHIO, BKMS_METABOLIC, PISTACHIO_RINGBREAKER, REAXYS, REAXYS_BIOCATALYSIS database, our tool offers high-accuracy predictions, reflecting the latest in chemical research and data.
Strategy Settings
Precursor scoring | Relevance Heuristic |
---|---|
Min. plausibility | 0.01 |
Model | Template_relevance |
Template Set | Pistachio/Bkms_metabolic/Pistachio_ringbreaker/Reaxys/Reaxys_biocatalysis |
Top-N result to add to graph | 6 |
Feasible Synthetic Routes
Q1: Why is LiTFSI considered a promising electrolyte salt for lithium-ion batteries?
A1: LiTFSI exhibits several advantageous properties over conventional lithium hexafluorophosphate (LiPF6), including:
- Higher thermal stability: This leads to improved safety and performance at elevated temperatures. [, ]
- Resistance to hydrolysis: This enhances moisture resistance, simplifying battery manufacturing and extending shelf life. []
- High ionic conductivity: LiTFSI-based electrolytes facilitate efficient ion transport, enabling high-rate performance. [, , , ]
Q2: How does LiTFSI interact with electrode materials like silicon and impact battery performance?
A3: In silicon anodes, the interaction between LiTFSI and the silicon surface can lead to the formation of an unstable solid electrolyte interphase (SEI), resulting in capacity fading. Researchers are exploring strategies like incorporating electrolyte additives and novel binders to mitigate these issues. [, ]
Q3: What strategies can mitigate aluminum corrosion caused by LiTFSI in high-voltage batteries?
A3: While LiTFSI offers benefits, it can corrode aluminum current collectors at high voltages. Strategies to circumvent this include:
- Low-voltage operation: Limiting the cell voltage below the threshold for aluminum corrosion. []
- Salt additives: Incorporating additives like lithium tetrafluoroborate (LiBF4) or lithium difluoro(oxalato)borate (LiDFOB) to suppress corrosion. []
Q4: What is the role of LiTFSI in gel polymer electrolytes (GPEs)?
A6: In GPEs, LiTFSI serves as the primary source of charge carriers. It dissolves in a polymer matrix and dissociates into Li+ and TFSI- ions, enabling ionic conductivity. The properties of the GPE, such as ionic conductivity and electrochemical stability, depend on the polymer-salt interactions and the concentration of LiTFSI. []
Q5: How does the presence of LiTFSI affect the polymerization of methyl methacrylate (MMA)?
A7: LiTFSI, typically considered a poor initiator for MMA polymerization, can be used in conjunction with fluoroethylene carbonate (FEC). FEC's high dielectric constant promotes LiTFSI dissociation, enhancing its ability to initiate MMA polymerization. This approach facilitates the creation of novel polymer electrolytes with desirable properties for lithium metal batteries. []
Q6: What is the molecular formula and weight of LiTFSI?
A6: The molecular formula of LiTFSI is C2F6LiNO4S2, and its molecular weight is 287.09 g/mol.
Q7: What spectroscopic techniques are commonly employed to characterize LiTFSI?
A7: Common techniques include:
- Fourier-transform infrared spectroscopy (FTIR): This method helps identify functional groups and analyze interactions between LiTFSI and other components in electrolytes. []
- Nuclear magnetic resonance (NMR) spectroscopy: NMR provides insights into the solvation structure and dynamics of LiTFSI in various solvents. []
- X-ray photoelectron spectroscopy (XPS): This surface-sensitive technique is valuable for analyzing the composition and chemical states of SEI layers formed in the presence of LiTFSI. [, ]
Q8: What is the role of additives like fluoroethylene carbonate (FEC) in LiTFSI-based electrolytes?
A11: FEC acts as a solid electrolyte interphase (SEI)-forming additive, contributing to a more stable and robust SEI layer on electrode surfaces. This, in turn, improves the cycling performance and lifespan of the battery. []
Q9: How does the thermal stability of LiTFSI contribute to battery safety?
A13: LiTFSI's higher thermal stability compared to LiPF6 reduces the risk of electrolyte decomposition and thermal runaway at elevated temperatures, contributing to safer battery operation. [, ]
Q10: Are there any environmental concerns associated with LiTFSI?
A14: While LiTFSI offers advantages over LiPF6, its potential environmental impact requires further investigation. Research on its biodegradability, ecotoxicological effects, and sustainable disposal methods is crucial. []
Q11: How is computational chemistry employed in LiTFSI research?
A11: Computational methods such as molecular dynamics (MD) simulations and density functional theory (DFT) calculations are essential for:
- Understanding ion transport mechanisms: Simulations provide insights into the movement of Li+ and TFSI- ions in different electrolyte systems. [, , ]
- Predicting electrolyte properties: Computational models help predict ionic conductivity, viscosity, and other critical parameters based on electrolyte composition. []
- Designing novel electrolyte materials: Simulations guide the development of new LiTFSI-based electrolytes with improved performance and safety characteristics. [, ]
Haftungsausschluss und Informationen zu In-Vitro-Forschungsprodukten
Bitte beachten Sie, dass alle Artikel und Produktinformationen, die auf BenchChem präsentiert werden, ausschließlich zu Informationszwecken bestimmt sind. Die auf BenchChem zum Kauf angebotenen Produkte sind speziell für In-vitro-Studien konzipiert, die außerhalb lebender Organismen durchgeführt werden. In-vitro-Studien, abgeleitet von dem lateinischen Begriff "in Glas", beinhalten Experimente, die in kontrollierten Laborumgebungen unter Verwendung von Zellen oder Geweben durchgeführt werden. Es ist wichtig zu beachten, dass diese Produkte nicht als Arzneimittel oder Medikamente eingestuft sind und keine Zulassung der FDA für die Vorbeugung, Behandlung oder Heilung von medizinischen Zuständen, Beschwerden oder Krankheiten erhalten haben. Wir müssen betonen, dass jede Form der körperlichen Einführung dieser Produkte in Menschen oder Tiere gesetzlich strikt untersagt ist. Es ist unerlässlich, sich an diese Richtlinien zu halten, um die Einhaltung rechtlicher und ethischer Standards in Forschung und Experiment zu gewährleisten.