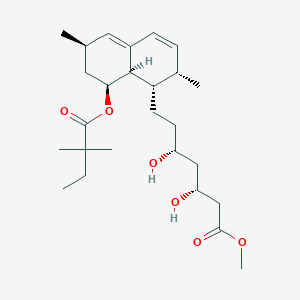
Simvastatin Hydroxy Acid Methyl Ester
Übersicht
Beschreibung
Simvastatin Hydroxy Acid Methyl Ester (SHAME) is a derivative of simvastatin, a widely prescribed statin used to lower cholesterol by inhibiting HMG-CoA reductase. SHAME is formed under specific chemical conditions, such as the alkaline methanolic treatment of simvastatin hydroxy acid (SVA), the active metabolite of simvastatin . Structurally, SHAME retains the core pharmacophore of simvastatin—a 3,5-dihydroxy acid motif—but features a methyl ester group at the hydroxy acid position, enhancing its lipophilicity compared to SVA .
SHAME is primarily studied as a degradation product or analytical reference standard. For instance, in pharmaceutical stability testing, SHAME formation is observed when simvastatin suspensions are treated with methanol under alkaline conditions, highlighting its role in overestimating degradation rates if improper solvents are used . Non-compendial reference standards like SHAME are critical for quantifying impurities in simvastatin formulations, validated via NMR, FT-IR, and mass spectrometry .
Vorbereitungsmethoden
Synthetic Routes and Reaction Conditions
Simvastatin Hydroxy Acid Methyl Ester is synthesized from Simvastatin through a series of chemical reactions. The primary synthetic route involves the hydrolysis of the lactone ring in Simvastatin to form Simvastatin Hydroxy Acid, followed by esterification to produce the methyl ester derivative . The reaction conditions typically involve acidic or basic hydrolysis followed by esterification using methanol in the presence of an acid catalyst .
Industrial Production Methods
Industrial production of this compound follows similar synthetic routes but on a larger scale. The process involves the use of large reactors and controlled conditions to ensure high yield and purity. The key steps include the hydrolysis of Simvastatin, purification of the hydroxy acid intermediate, and subsequent esterification .
Analyse Chemischer Reaktionen
Hydrolysis Reactions
SHAME undergoes hydrolysis under varying conditions to form pharmacologically active or inactive metabolites:
Acidic Hydrolysis
In acidic environments (e.g., HCl), SHAME’s lactone ring opens to form the corresponding β,δ-dihydroxy acid. This reaction is critical for its activation in vivo .
Basic Hydrolysis
Treatment with NaOH (2N) at reflux converts SHAME to simvastatin β-hydroxy acid (BHA), its active metabolite, via saponification of the methyl ester group . This reaction is pH-dependent and yields >85% BHA under optimized conditions .
Reaction Scheme :
Enzymatic Hydrolysis
-
Esterases : Carboxylesterases in the liver and plasma hydrolyze SHAME to BHA, enhancing its bioavailability .
-
CYP450 Metabolism : CYP3A4/5 mediates oxidative metabolism, producing 6'-hydroxy and 6'-exomethylene derivatives .
HMGylation and Post-Translational Modifications
SHAME’s inhibition of HMG-CoA reductase induces a unique post-translational modification termed HMGylation , where HMG groups (3-hydroxy-3-methylglutaryl) covalently bind to proteins :
Thioester and Ester Bond Formation
Key Finding :
HMGylation of fatty acid synthase (FAS) occurs near HMG-CoA reductase in the endoplasmic reticulum, confirmed via proximity ligation assays . This modification is reversible upon statin withdrawal .
Stability Under Forced Degradation Conditions
Forced degradation studies reveal SHAME’s susceptibility to environmental factors :
Condition | Degradation Products | % Degradation |
---|---|---|
Acidic (0.1N HCl, 1h) | Hydroxyl acid derivatives | 15.2% |
Basic (0.005N NaOH, 1h) | β-hydroxy acid (BHA) | 87.0% |
Oxidative (3% H₂O₂, 48h) | Oxidized metabolites | 1.86% |
Thermal (60°C, 5 days) | None | <0.5% |
Key Synthetic Steps
-
Enzymatic Hydrolysis : Lovastatin is hydrolyzed to triol acid using carboxylesterases .
-
Lactonization : Triol acid cyclizes to diol lactone under acidic conditions .
-
Regioselective Acylation : Enzymatic acylation (e.g., acetyltransferases) introduces methyl ester groups .
Biotransformation in Probiotic Bacteria
SHAME is biotransformed by Lactobacillus spp. via esterase activity, reducing total drug levels by 34% in 48 hours .
Interaction with Reactive Oxygen Species (ROS)
In ischemia-reperfusion injury models, SHAME reduces oxidative stress by:
Mechanism :
Comparative Reactivity with Other Statins
Wissenschaftliche Forschungsanwendungen
Lipid Regulation
Simvastatin Hydroxy Acid Methyl Ester plays a crucial role in managing hyperlipidemia. It inhibits the HMG-CoA reductase enzyme, leading to decreased cholesterol synthesis in the liver. This results in:
- Lowered levels of total cholesterol and low-density lipoprotein cholesterol (LDL-C).
- Increased high-density lipoprotein cholesterol (HDL-C) levels.
- Reduced triglyceride concentrations .
The compound is indicated for various conditions, including:
- Primary hyperlipidemia (Fredrickson type IIa).
- Mixed dyslipidemia (Fredrickson type IIb).
- Hypertriglyceridemia (Fredrickson type IV) .
Cardiovascular Disease Prevention
This compound has been extensively studied for its cardioprotective effects. It not only lowers lipid levels but also:
- Reduces cardiovascular morbidity and mortality.
- Is recommended for patients at high risk of coronary events due to existing cardiovascular conditions .
Anti-Inflammatory Properties
Recent studies highlight the anti-inflammatory effects of simvastatin, which extend beyond its lipid-lowering capabilities. Research indicates that simvastatin can:
- Significantly reduce inflammation in models such as carrageenan-induced edema.
- Exhibit comparable efficacy to traditional anti-inflammatory drugs like indomethacin .
In murine models, simvastatin treatment resulted in:
- Decreased aortic cholesterol accumulation without altering plasma lipid levels.
- A reduction in atherosclerotic plaque formation, suggesting a direct antiatherosclerotic effect independent of lipid modulation .
Biotransformation and Probiotic Interaction
Recent investigations into the biotransformation of simvastatin by probiotic bacteria reveal:
- Enhanced bioaccumulation of simvastatin within bacterial cells when exposed to bile acids.
- Potential metabolic pathways involving ester hydrolysis and hydroxylation of the lactone ring structure .
This interaction suggests that gut microbiota may influence the pharmacokinetics and efficacy of simvastatin therapy, highlighting the importance of personalized medicine approaches .
Case Studies and Clinical Trials
Numerous clinical trials have assessed the efficacy of simvastatin and its derivatives. For instance:
- The Heart Protection Study demonstrated a significant reduction in major vascular events among participants treated with simvastatin compared to placebo .
- Other studies have focused on specific populations, such as adolescents with familial hypercholesterolemia, showing positive outcomes in lipid profile management while monitoring safety profiles .
Wirkmechanismus
Simvastatin Hydroxy Acid Methyl Ester exerts its effects by inhibiting the enzyme hydroxymethylglutaryl-coenzyme A reductase. This enzyme catalyzes the conversion of hydroxymethylglutaryl-coenzyme A to mevalonate, a key step in the biosynthesis of cholesterol . By inhibiting this enzyme, the compound effectively reduces the production of cholesterol in the liver, leading to lower blood cholesterol levels .
Vergleich Mit ähnlichen Verbindungen
Structural and Metabolic Differences
Table 1: Structural and Metabolic Features of SHAME and Related Compounds
Hydrolysis and Esterase Sensitivity
- SHAME vs. SVA: SHAME’s methyl ester group reduces susceptibility to esterase-mediated hydrolysis compared to SVA. In vitro studies show SHAME formation is negligible when acetonitrile replaces methanol, emphasizing solvent-dependent stability .
- Simvastatin vs. Lovastatin : Simvastatin’s additional methyl group on the butyrate ester creates steric hindrance, slowing hydrolysis by plasma/liver esterases. Lovastatin hydrolyzes 1.5–2x faster, producing LVA more rapidly . This structural difference increases simvastatin’s bioavailability and duration of action .
Enzymatic Pathways and Metabolite Activity
- CYP3A4 Metabolism: Both simvastatin and lovastatin undergo CYP3A4-mediated oxidation, but simvastatin generates more stable metabolites (e.g., 6′-OH-SV) with prolonged HMG-CoA reductase inhibition .
- Esterase Activity : SHAME resists esterase cleavage, unlike SVA, which rapidly converts back to the lactone form in acidic conditions . Lovastatin’s lack of a methyl group makes its ester bond more vulnerable to hydrolysis, explaining its shorter half-life .
Pharmacological Implications
- Efficacy : Simvastatin’s slower hydrolysis and higher bioavailability translate to greater LDL cholesterol reduction compared to lovastatin . SHAME’s stability under alkaline conditions makes it a useful analytical marker but irrelevant therapeutically .
- Adverse Effects : SVA’s hydroxy acid form directly activates skeletal muscle RyR1 channels, contributing to statin-induced myopathy. SHAME’s esterified structure likely mitigates this interaction .
Key Research Findings
Table 2: Comparative Hydrolysis Rates in Human Plasma (Normalized to Simvastatin = 1.0)
- Gender Differences : Females exhibit 20–30% faster hydrolysis of simvastatin to SVA than males, linked to higher esterase activity .
- Species Variability : Rodents metabolize simvastatin faster than humans due to higher plasma esterase activity, necessitating cautious extrapolation of preclinical data .
Biologische Aktivität
Simvastatin Hydroxy Acid Methyl Ester (SHAME) is an active metabolite of simvastatin, a widely used statin for lowering cholesterol levels. This article explores the biological activity of SHAME, focusing on its pharmacological effects, mechanisms of action, and implications for various health conditions.
Overview of Simvastatin and Its Metabolites
Simvastatin is an HMG-CoA reductase inhibitor that primarily acts in the liver to reduce cholesterol synthesis. It is administered as a prodrug that is converted into its active form, β-hydroxyacid, through hydrolysis and metabolic activation. The hydroxy acid form is crucial for the inhibition of HMG-CoA reductase, which is a key enzyme in the mevalonate pathway responsible for cholesterol production .
Key Metabolites :
- β-Hydroxyacid : The primary active form responsible for lipid-lowering effects.
- 6'-Hydroxy and other derivatives : These metabolites also exhibit biological activity but are less studied compared to the β-hydroxyacid .
This compound exerts its biological effects through several mechanisms:
- Cholesterol Lowering : By inhibiting HMG-CoA reductase, SHAME decreases the synthesis of cholesterol in the liver, leading to increased uptake of low-density lipoprotein (LDL) from the bloodstream .
- Pleiotropic Effects : Beyond lipid-lowering, SHAME exhibits various pleiotropic effects:
- Impact on Lipidomic Profiles : Research indicates that simvastatin can alter lipidomic markers in liver cells, affecting fatty acid synthesis pathways and influencing overall lipid metabolism .
1. Anti-Atherogenic Effects
A study involving mice deficient in apolipoprotein E demonstrated that simvastatin significantly reduced total cholesterol content in the aorta by approximately 23%, indicating its potential anti-atherogenic properties even without altering plasma lipid levels .
Parameter | Control Group | Simvastatin Group |
---|---|---|
Total Cholesterol (nmol/mg wet wt) | 56 ± 4 | 43 ± 3 |
Free Cholesterol (nmol/mg) | 38 ± 2 | 31 ± 2 |
Cholesteryl Ester (nmol/mg) | 17 ± 2 | 11 ± 1 |
2. Transgenerational Effects
A case study on the amphipod Gammarus locusta revealed transgenerational reproductive effects due to exposure to simvastatin. This research highlights potential long-term ecological impacts of pharmaceuticals, suggesting that simvastatin may disrupt reproductive health across generations .
3. Bone Health
Several studies have indicated that simvastatin promotes bone healing and may have therapeutic applications in osteoporosis. Its role in enhancing bone metabolism suggests additional benefits beyond cardiovascular health .
Pharmacokinetics and Safety Profile
Simvastatin undergoes extensive first-pass metabolism in the liver, with CYP3A4 being the primary enzyme involved. The pharmacokinetics reveal a Tmax (time to reach maximum concentration) of approximately 1.44 hours and a half-life of about 4.85 hours .
While generally well-tolerated, potential side effects include muscle pain and liver enzyme elevations. Genetic polymorphisms in CYP3A5 can influence individual responses to simvastatin therapy, necessitating personalized approaches to treatment .
Q & A
Basic Research Questions
Q. What are the validated analytical methods for quantifying SIMA and its prodrug simvastatin lactone in biological matrices?
Methodological Answer:
- Use HPLC-MS/MS with isotope-labeled internal standards (e.g., simvastatin-d6 and SIMA-d6) for precise quantification. Plasma, bile, and tissue homogenates should be prepared with 100 mM ammonium acetate (pH 4.5) and extracted via solid-phase extraction (e.g., Oasis HLB cartridges). Calibration curves must cover expected concentration ranges (e.g., 0.1–1000 ng/mL), with validation for specificity, accuracy (85–115%), and precision (CV <15%) .
- Reference USP monographs for chromatographic conditions, such as C18 columns and mobile phases combining acetonitrile with acidic buffers (e.g., 0.1% formic acid) .
Q. How is SIMA synthesized from simvastatin lactone, and what purity controls are required?
Methodological Answer:
- Simvastatin lactone is hydrolyzed enzymatically (e.g., using Cyp2c11/Cyp3a isoforms in rats) or non-enzymatically (via esterases/paraoxonases). Validate conversion efficiency using NMR or HPLC to confirm >98% purity.
- For synthetic routes, reduce lovastatin with lithium hydroxide to yield dihydroxy intermediates, followed by methyl esterification. Monitor reaction progress with TLC (silica gel, ethyl acetate/hexane) and confirm final structure via FT-IR (C=O ester bands) and mass spectrometry .
Advanced Research Questions
Q. How does nonalcoholic steatohepatitis (NASH) alter SIMA pharmacokinetics, and what experimental models are appropriate to study this?
Methodological Answer:
- Use methionine/choline-deficient (MCD) diet-induced NASH in Sprague-Dawley rats. After 8 weeks, administer SIM lactone (1 mg/kg intra-arterially) and collect plasma, bile, and tissues (liver, kidney) at timed intervals. Measure SIMA exposure via AUC and Cmax, noting reduced hepatic OATP1a/1b expression via qPCR or Western blot .
- Address compensatory transport mechanisms by comparing wild-type vs. OATP1a/1b cluster knockout models to isolate transporter-specific effects .
Q. How should researchers resolve contradictory data on SIMA’s metabolic pathways (enzymatic vs. non-enzymatic hydrolysis)?
Methodological Answer:
- Conduct dual-pathway inhibition studies: (1) Use Cyp3a/Cyp2c11 inhibitors (e.g., ketoconazole) to block enzymatic hydrolysis; (2) Apply esterase inhibitors (e.g., paraoxon) to suppress non-enzymatic pathways. Compare SIMA formation rates in plasma and liver microsomes via LC-MS.
- Statistical analysis: Use two-way ANOVA to assess interactions between pathways and Tukey’s post-hoc test for group comparisons. Report effect sizes and confidence intervals .
Q. What in vivo experimental designs minimize variability in SIMA biliary excretion studies?
Methodological Answer:
- Cannulate the common bile duct under urethane anesthesia (1.2 g/kg) and maintain body temperature at 37°C. Collect bile at 15-minute intervals post-dosing. Normalize excretion rates to body weight and liver mass.
- Control for diet-induced variability: Pair-fed control groups must receive methionine/choline-replete diets. Validate NASH progression via histopathology (e.g., hematoxylin/eosin staining for steatosis) .
Q. Methodological Best Practices
Q. How to ensure reproducibility in SIMA metabolism studies across laboratories?
Methodological Answer:
- Adhere to the ARRIVE guidelines for in vivo experiments: Report animal strain, diet composition (e.g., Dyets Inc. MCD diet), and anesthesia protocols. Share raw pharmacokinetic data (e.g., plasma SIMA concentrations) in public repositories like Figshare.
- Include detailed Supplementary Materials with HPLC gradients, primer sequences for OATP quantification, and statistical code (R/Python scripts) .
Q. What statistical approaches are recommended for pharmacokinetic modeling of SIMA exposure?
Methodological Answer:
- Use non-compartmental analysis (NCA) for AUC and half-life calculations (e.g., Phoenix WinNonlin). For compartmental modeling, apply Bayesian hierarchical models to account for inter-individual variability.
- Validate models with goodness-of-fit plots (observed vs. predicted concentrations) and bootstrap resampling (n=1000) to estimate parameter confidence intervals .
Q. Safety and Compliance
Q. What safety protocols are critical when handling SIMA in preclinical studies?
Methodological Answer:
- Follow GHS guidelines: Use PPE (gloves, lab coats) and chemical fume hoods for weighing/powder handling. Store SIMA in desiccators at -20°C to prevent hydrolysis.
- For in vivo dosing, prepare lactone formulations in 10% ethanol/45% propylene glycol to enhance solubility. Document adverse events (e.g., myopathy) via serum creatine kinase assays .
Eigenschaften
IUPAC Name |
methyl (3R,5R)-7-[(1S,2S,6R,8S,8aR)-8-(2,2-dimethylbutanoyloxy)-2,6-dimethyl-1,2,6,7,8,8a-hexahydronaphthalen-1-yl]-3,5-dihydroxyheptanoate | |
---|---|---|
Source | PubChem | |
URL | https://pubchem.ncbi.nlm.nih.gov | |
Description | Data deposited in or computed by PubChem | |
InChI |
InChI=1S/C26H42O6/c1-7-26(4,5)25(30)32-22-13-16(2)12-18-9-8-17(3)21(24(18)22)11-10-19(27)14-20(28)15-23(29)31-6/h8-9,12,16-17,19-22,24,27-28H,7,10-11,13-15H2,1-6H3/t16-,17-,19+,20+,21-,22-,24-/m0/s1 | |
Source | PubChem | |
URL | https://pubchem.ncbi.nlm.nih.gov | |
Description | Data deposited in or computed by PubChem | |
InChI Key |
ANVXHKJGHXLLPK-RVTXAWHXSA-N | |
Source | PubChem | |
URL | https://pubchem.ncbi.nlm.nih.gov | |
Description | Data deposited in or computed by PubChem | |
Canonical SMILES |
CCC(C)(C)C(=O)OC1CC(C=C2C1C(C(C=C2)C)CCC(CC(CC(=O)OC)O)O)C | |
Source | PubChem | |
URL | https://pubchem.ncbi.nlm.nih.gov | |
Description | Data deposited in or computed by PubChem | |
Isomeric SMILES |
CCC(C)(C)C(=O)O[C@H]1C[C@H](C=C2[C@H]1[C@H]([C@H](C=C2)C)CC[C@H](C[C@H](CC(=O)OC)O)O)C | |
Source | PubChem | |
URL | https://pubchem.ncbi.nlm.nih.gov | |
Description | Data deposited in or computed by PubChem | |
Molecular Formula |
C26H42O6 | |
Source | PubChem | |
URL | https://pubchem.ncbi.nlm.nih.gov | |
Description | Data deposited in or computed by PubChem | |
DSSTOX Substance ID |
DTXSID70453246 | |
Record name | Simvastatin Hydroxy Acid Methyl Ester | |
Source | EPA DSSTox | |
URL | https://comptox.epa.gov/dashboard/DTXSID70453246 | |
Description | DSSTox provides a high quality public chemistry resource for supporting improved predictive toxicology. | |
Molecular Weight |
450.6 g/mol | |
Source | PubChem | |
URL | https://pubchem.ncbi.nlm.nih.gov | |
Description | Data deposited in or computed by PubChem | |
CAS No. |
145576-26-7 | |
Record name | Simvastatin Hydroxy Acid Methyl Ester | |
Source | EPA DSSTox | |
URL | https://comptox.epa.gov/dashboard/DTXSID70453246 | |
Description | DSSTox provides a high quality public chemistry resource for supporting improved predictive toxicology. | |
Haftungsausschluss und Informationen zu In-Vitro-Forschungsprodukten
Bitte beachten Sie, dass alle Artikel und Produktinformationen, die auf BenchChem präsentiert werden, ausschließlich zu Informationszwecken bestimmt sind. Die auf BenchChem zum Kauf angebotenen Produkte sind speziell für In-vitro-Studien konzipiert, die außerhalb lebender Organismen durchgeführt werden. In-vitro-Studien, abgeleitet von dem lateinischen Begriff "in Glas", beinhalten Experimente, die in kontrollierten Laborumgebungen unter Verwendung von Zellen oder Geweben durchgeführt werden. Es ist wichtig zu beachten, dass diese Produkte nicht als Arzneimittel oder Medikamente eingestuft sind und keine Zulassung der FDA für die Vorbeugung, Behandlung oder Heilung von medizinischen Zuständen, Beschwerden oder Krankheiten erhalten haben. Wir müssen betonen, dass jede Form der körperlichen Einführung dieser Produkte in Menschen oder Tiere gesetzlich strikt untersagt ist. Es ist unerlässlich, sich an diese Richtlinien zu halten, um die Einhaltung rechtlicher und ethischer Standards in Forschung und Experiment zu gewährleisten.