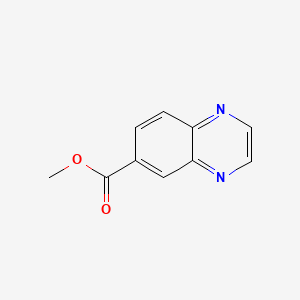
Methyl quinoxaline-6-carboxylate
Übersicht
Beschreibung
“Methyl quinoxaline-6-carboxylate” is a chemical compound with the empirical formula C10H8N2O2 . It is also known as "6-Quinoxalinecarboxylic acid methyl ester" .
Molecular Structure Analysis
The molecular weight of “Methyl quinoxaline-6-carboxylate” is 188.18 . The structure of quinoxaline is defined as a weakly basic bi-cyclic compound C8H6N2, having fused benzene and pyrazine rings .
Chemical Reactions Analysis
Quinoxalines have become a subject of extensive research due to their wide range of physicochemical and biological activities . They are used in the design and development of numerous bioactive molecules, dyes, fluorescent materials, electroluminescent materials, and organic sensitizers for solar cell applications and polymeric optoelectronic materials .
Physical And Chemical Properties Analysis
“Methyl quinoxaline-6-carboxylate” has a molecular weight of 188.18 . It has a density of 1.2±0.1 g/cm3, a boiling point of 315.1±15.0 °C at 760 mmHg, and a flash point of 144.4±20.4 °C .
Wissenschaftliche Forschungsanwendungen
Antimicrobial Activity
Methyl quinoxaline derivatives have been studied for their antimicrobial properties. They are known to be effective against a range of pathogenic bacteria and fungi. The compound’s structure allows it to interact with microbial cell walls, disrupting their integrity and leading to cell death .
Antituberculosis Activity
Tuberculosis, caused by Mycobacterium tuberculosis, remains a significant global health challenge. Methyl quinoxaline-6-carboxylate derivatives have shown promise in inhibiting the growth of this bacterium, offering potential as a novel treatment option .
Anticancer Properties
Quinoxaline derivatives are being explored for their potential use in cancer therapy. Their ability to interfere with DNA replication and cell division makes them suitable candidates for anticancer drugs. Research is ongoing to determine the efficacy and safety of these compounds in cancer treatment .
Antiviral Applications
The structural versatility of quinoxaline derivatives allows them to be tailored for antiviral activity. They can be designed to inhibit viral entry or replication, which is crucial in the development of new therapies for viral infections .
Anti-inflammatory Uses
Inflammation is a biological response to harmful stimuli, and excessive inflammation can lead to various diseases. Methyl quinoxaline derivatives have shown anti-inflammatory effects, which could be beneficial in treating conditions like arthritis and asthma .
Antioxidant Effects
Oxidative stress is implicated in many chronic diseases. Methyl quinoxaline derivatives exhibit antioxidant properties, neutralizing free radicals and potentially preventing or mitigating oxidative damage .
Antileishmanial Activity
Leishmaniasis is a disease caused by protozoan parasites. Some methyl quinoxaline derivatives have demonstrated activity against leishmanial species, suggesting their use in developing new antileishmanial agents .
Antimalarial Potential
Malaria is a life-threatening disease caused by Plasmodium parasites. Quinoxaline derivatives have been investigated for their antimalarial properties, with some compounds showing potent activity against strains of the malaria parasite .
Zukünftige Richtungen
Quinoxalines have become a subject of extensive research due to their emergence as an important chemical moiety . They have several prominent pharmacological effects and diverse therapeutic uses . Due to the current pandemic situation caused by SARS-COVID 19, it has become essential to synthesize drugs to combat deadly pathogens (bacteria, fungi, viruses) for now and near future . Therefore, “Methyl quinoxaline-6-carboxylate” and other quinoxalines have a promising future in medicinal chemistry .
Eigenschaften
IUPAC Name |
methyl quinoxaline-6-carboxylate | |
---|---|---|
Source | PubChem | |
URL | https://pubchem.ncbi.nlm.nih.gov | |
Description | Data deposited in or computed by PubChem | |
InChI |
InChI=1S/C10H8N2O2/c1-14-10(13)7-2-3-8-9(6-7)12-5-4-11-8/h2-6H,1H3 | |
Source | PubChem | |
URL | https://pubchem.ncbi.nlm.nih.gov | |
Description | Data deposited in or computed by PubChem | |
InChI Key |
VFPWJISMXACHIG-UHFFFAOYSA-N | |
Source | PubChem | |
URL | https://pubchem.ncbi.nlm.nih.gov | |
Description | Data deposited in or computed by PubChem | |
Canonical SMILES |
COC(=O)C1=CC2=NC=CN=C2C=C1 | |
Source | PubChem | |
URL | https://pubchem.ncbi.nlm.nih.gov | |
Description | Data deposited in or computed by PubChem | |
Molecular Formula |
C10H8N2O2 | |
Source | PubChem | |
URL | https://pubchem.ncbi.nlm.nih.gov | |
Description | Data deposited in or computed by PubChem | |
DSSTOX Substance ID |
DTXSID60381761 | |
Record name | methyl quinoxaline-6-carboxylate | |
Source | EPA DSSTox | |
URL | https://comptox.epa.gov/dashboard/DTXSID60381761 | |
Description | DSSTox provides a high quality public chemistry resource for supporting improved predictive toxicology. | |
Molecular Weight |
188.18 g/mol | |
Source | PubChem | |
URL | https://pubchem.ncbi.nlm.nih.gov | |
Description | Data deposited in or computed by PubChem | |
Solubility |
22.7 [ug/mL] (The mean of the results at pH 7.4) | |
Record name | SID26729613 | |
Source | Burnham Center for Chemical Genomics | |
URL | https://pubchem.ncbi.nlm.nih.gov/bioassay/1996#section=Data-Table | |
Description | Aqueous solubility in buffer at pH 7.4 | |
Product Name |
Methyl quinoxaline-6-carboxylate | |
CAS RN |
23088-23-5 | |
Record name | methyl quinoxaline-6-carboxylate | |
Source | EPA DSSTox | |
URL | https://comptox.epa.gov/dashboard/DTXSID60381761 | |
Description | DSSTox provides a high quality public chemistry resource for supporting improved predictive toxicology. | |
Record name | Methyl 6-quinoxalinecarboxylate | |
Source | European Chemicals Agency (ECHA) | |
URL | https://echa.europa.eu/information-on-chemicals | |
Description | The European Chemicals Agency (ECHA) is an agency of the European Union which is the driving force among regulatory authorities in implementing the EU's groundbreaking chemicals legislation for the benefit of human health and the environment as well as for innovation and competitiveness. | |
Explanation | Use of the information, documents and data from the ECHA website is subject to the terms and conditions of this Legal Notice, and subject to other binding limitations provided for under applicable law, the information, documents and data made available on the ECHA website may be reproduced, distributed and/or used, totally or in part, for non-commercial purposes provided that ECHA is acknowledged as the source: "Source: European Chemicals Agency, http://echa.europa.eu/". Such acknowledgement must be included in each copy of the material. ECHA permits and encourages organisations and individuals to create links to the ECHA website under the following cumulative conditions: Links can only be made to webpages that provide a link to the Legal Notice page. | |
Synthesis routes and methods
Procedure details
Retrosynthesis Analysis
AI-Powered Synthesis Planning: Our tool employs the Template_relevance Pistachio, Template_relevance Bkms_metabolic, Template_relevance Pistachio_ringbreaker, Template_relevance Reaxys, Template_relevance Reaxys_biocatalysis model, leveraging a vast database of chemical reactions to predict feasible synthetic routes.
One-Step Synthesis Focus: Specifically designed for one-step synthesis, it provides concise and direct routes for your target compounds, streamlining the synthesis process.
Accurate Predictions: Utilizing the extensive PISTACHIO, BKMS_METABOLIC, PISTACHIO_RINGBREAKER, REAXYS, REAXYS_BIOCATALYSIS database, our tool offers high-accuracy predictions, reflecting the latest in chemical research and data.
Strategy Settings
Precursor scoring | Relevance Heuristic |
---|---|
Min. plausibility | 0.01 |
Model | Template_relevance |
Template Set | Pistachio/Bkms_metabolic/Pistachio_ringbreaker/Reaxys/Reaxys_biocatalysis |
Top-N result to add to graph | 6 |
Feasible Synthetic Routes
Haftungsausschluss und Informationen zu In-Vitro-Forschungsprodukten
Bitte beachten Sie, dass alle Artikel und Produktinformationen, die auf BenchChem präsentiert werden, ausschließlich zu Informationszwecken bestimmt sind. Die auf BenchChem zum Kauf angebotenen Produkte sind speziell für In-vitro-Studien konzipiert, die außerhalb lebender Organismen durchgeführt werden. In-vitro-Studien, abgeleitet von dem lateinischen Begriff "in Glas", beinhalten Experimente, die in kontrollierten Laborumgebungen unter Verwendung von Zellen oder Geweben durchgeführt werden. Es ist wichtig zu beachten, dass diese Produkte nicht als Arzneimittel oder Medikamente eingestuft sind und keine Zulassung der FDA für die Vorbeugung, Behandlung oder Heilung von medizinischen Zuständen, Beschwerden oder Krankheiten erhalten haben. Wir müssen betonen, dass jede Form der körperlichen Einführung dieser Produkte in Menschen oder Tiere gesetzlich strikt untersagt ist. Es ist unerlässlich, sich an diese Richtlinien zu halten, um die Einhaltung rechtlicher und ethischer Standards in Forschung und Experiment zu gewährleisten.