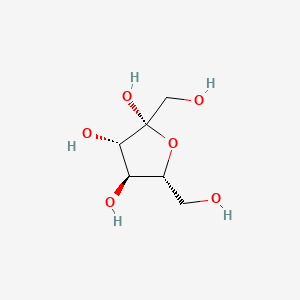
beta-D-fructofuranose
Übersicht
Beschreibung
Beta-D-fructofuranose is a carbohydrate derivative that includes a five-membered ring system consisting of four carbon atoms and one oxygen atom . It contains a total of 24 bonds, including 12 non-H bonds, 2 rotatable bonds, 1 five-membered ring, 5 hydroxyl groups, 2 primary alcohols, 2 secondary alcohols, 1 ether (aliphatic), and 1 Oxolane .
Synthesis Analysis
The production of fructo-oligosaccharides (FOS) involves transfructosylation reactions where fructosyltransferases act as biocatalysts . The enzyme β-fructofuranosidase from the yeast Rhodotorula dairenensis has been characterized biochemically . This enzyme shows broad substrate specificity, hydrolyzing sucrose, 1-kestose, nystose, leucrose, raffinose, and inulin .Molecular Structure Analysis
The molecular mass of the enzyme β-fructofuranose was estimated to be 680 kDa by analytical gel filtration and 172 kDa by sodium dodecyl sulfate-polyacrylamide gel electrophoresis . The N-linked carbohydrate accounts for 16% of the total mass .Wissenschaftliche Forschungsanwendungen
Nutrition Improvement
Beta-D-fructofuranose is used in the food industry to improve nutrition. It is applied to burdock root to increase functional substances . The enzyme DFA-IIIase from Duffyella gerundensis A4 is used to process burdock, increasing the production of inulobiose, a prebiotic fructo-oligosaccharide . This process utilizes the polysaccharide inulin to improve nutrition and health .
Prebiotic Properties
Beta-D-fructofuranose has prebiotic properties. It is a component of fructo- and galacto-oligosaccharides (FOS and GOS), which are non-digestible oligosaccharides with prebiotic properties . These oligosaccharides can be incorporated into a wide number of products .
Low Caloric Sweeteners
FOS and GOS, which contain beta-D-fructofuranose, are low caloric sweeteners . They contribute to body weight control and give a feeling of satiety .
Constipation Relief
Beta-D-fructofuranose, as a component of FOS and GOS, can help relieve constipation .
Low Glycemic Index
FOS and GOS have a low glycemic index . This makes them suitable for consumption by individuals with diabetes .
Non-cariogenic
Beta-D-fructofuranose, as a part of FOS and GOS, is not cariogenic . This means it does not contribute to tooth decay .
Safety And Hazards
Zukünftige Richtungen
Fructo- and galacto-oligosaccharides (FOS and GOS) are widely known because of their prebiotic properties. They are low caloric sweeteners, give a feeling of satiety, contribute to body weight control, relieve constipation, have a low glycemic index, and are not cariogenic . The likelihood of allergic reactions to the β‐fructofuranosidase from S. cerevisiae strain INV is expected not to exceed the likelihood of allergic reactions to tomato .
Eigenschaften
IUPAC Name |
(2R,3S,4S,5R)-2,5-bis(hydroxymethyl)oxolane-2,3,4-triol | |
---|---|---|
Source | PubChem | |
URL | https://pubchem.ncbi.nlm.nih.gov | |
Description | Data deposited in or computed by PubChem | |
InChI |
InChI=1S/C6H12O6/c7-1-3-4(9)5(10)6(11,2-8)12-3/h3-5,7-11H,1-2H2/t3-,4-,5+,6-/m1/s1 | |
Source | PubChem | |
URL | https://pubchem.ncbi.nlm.nih.gov | |
Description | Data deposited in or computed by PubChem | |
InChI Key |
RFSUNEUAIZKAJO-ARQDHWQXSA-N | |
Source | PubChem | |
URL | https://pubchem.ncbi.nlm.nih.gov | |
Description | Data deposited in or computed by PubChem | |
Canonical SMILES |
C(C1C(C(C(O1)(CO)O)O)O)O | |
Source | PubChem | |
URL | https://pubchem.ncbi.nlm.nih.gov | |
Description | Data deposited in or computed by PubChem | |
Isomeric SMILES |
C([C@@H]1[C@H]([C@@H]([C@](O1)(CO)O)O)O)O | |
Source | PubChem | |
URL | https://pubchem.ncbi.nlm.nih.gov | |
Description | Data deposited in or computed by PubChem | |
Molecular Formula |
C6H12O6 | |
Source | PubChem | |
URL | https://pubchem.ncbi.nlm.nih.gov | |
Description | Data deposited in or computed by PubChem | |
DSSTOX Substance ID |
DTXSID60859011 | |
Record name | beta-D-Fructofuranose | |
Source | EPA DSSTox | |
URL | https://comptox.epa.gov/dashboard/DTXSID60859011 | |
Description | DSSTox provides a high quality public chemistry resource for supporting improved predictive toxicology. | |
Molecular Weight |
180.16 g/mol | |
Source | PubChem | |
URL | https://pubchem.ncbi.nlm.nih.gov | |
Description | Data deposited in or computed by PubChem | |
Physical Description |
Solid | |
Record name | D-Fructose | |
Source | Human Metabolome Database (HMDB) | |
URL | http://www.hmdb.ca/metabolites/HMDB0000660 | |
Description | The Human Metabolome Database (HMDB) is a freely available electronic database containing detailed information about small molecule metabolites found in the human body. | |
Explanation | HMDB is offered to the public as a freely available resource. Use and re-distribution of the data, in whole or in part, for commercial purposes requires explicit permission of the authors and explicit acknowledgment of the source material (HMDB) and the original publication (see the HMDB citing page). We ask that users who download significant portions of the database cite the HMDB paper in any resulting publications. | |
Solubility |
778 mg/mL at 20 °C | |
Record name | D-Fructose | |
Source | Human Metabolome Database (HMDB) | |
URL | http://www.hmdb.ca/metabolites/HMDB0000660 | |
Description | The Human Metabolome Database (HMDB) is a freely available electronic database containing detailed information about small molecule metabolites found in the human body. | |
Explanation | HMDB is offered to the public as a freely available resource. Use and re-distribution of the data, in whole or in part, for commercial purposes requires explicit permission of the authors and explicit acknowledgment of the source material (HMDB) and the original publication (see the HMDB citing page). We ask that users who download significant portions of the database cite the HMDB paper in any resulting publications. | |
Product Name |
beta-D-fructofuranose | |
CAS RN |
470-23-5, 53188-23-1, 57-48-7 | |
Record name | β-D-Fructofuranose | |
Source | CAS Common Chemistry | |
URL | https://commonchemistry.cas.org/detail?cas_rn=470-23-5 | |
Description | CAS Common Chemistry is an open community resource for accessing chemical information. Nearly 500,000 chemical substances from CAS REGISTRY cover areas of community interest, including common and frequently regulated chemicals, and those relevant to high school and undergraduate chemistry classes. This chemical information, curated by our expert scientists, is provided in alignment with our mission as a division of the American Chemical Society. | |
Explanation | The data from CAS Common Chemistry is provided under a CC-BY-NC 4.0 license, unless otherwise stated. | |
Record name | beta-d-Fructofuranose | |
Source | ChemIDplus | |
URL | https://pubchem.ncbi.nlm.nih.gov/substance/?source=chemidplus&sourceid=0053188231 | |
Description | ChemIDplus is a free, web search system that provides access to the structure and nomenclature authority files used for the identification of chemical substances cited in National Library of Medicine (NLM) databases, including the TOXNET system. | |
Record name | D-fructose | |
Source | DTP/NCI | |
URL | https://dtp.cancer.gov/dtpstandard/servlet/dwindex?searchtype=NSC&outputformat=html&searchlist=760385 | |
Description | The NCI Development Therapeutics Program (DTP) provides services and resources to the academic and private-sector research communities worldwide to facilitate the discovery and development of new cancer therapeutic agents. | |
Explanation | Unless otherwise indicated, all text within NCI products is free of copyright and may be reused without our permission. Credit the National Cancer Institute as the source. | |
Record name | beta-D-Fructofuranose | |
Source | EPA DSSTox | |
URL | https://comptox.epa.gov/dashboard/DTXSID60859011 | |
Description | DSSTox provides a high quality public chemistry resource for supporting improved predictive toxicology. | |
Record name | .BETA.-D.-FRUCTOFURANOSE | |
Source | FDA Global Substance Registration System (GSRS) | |
URL | https://gsrs.ncats.nih.gov/ginas/app/beta/substances/B986VTP17J | |
Description | The FDA Global Substance Registration System (GSRS) enables the efficient and accurate exchange of information on what substances are in regulated products. Instead of relying on names, which vary across regulatory domains, countries, and regions, the GSRS knowledge base makes it possible for substances to be defined by standardized, scientific descriptions. | |
Explanation | Unless otherwise noted, the contents of the FDA website (www.fda.gov), both text and graphics, are not copyrighted. They are in the public domain and may be republished, reprinted and otherwise used freely by anyone without the need to obtain permission from FDA. Credit to the U.S. Food and Drug Administration as the source is appreciated but not required. | |
Record name | D-Fructose | |
Source | Human Metabolome Database (HMDB) | |
URL | http://www.hmdb.ca/metabolites/HMDB0000660 | |
Description | The Human Metabolome Database (HMDB) is a freely available electronic database containing detailed information about small molecule metabolites found in the human body. | |
Explanation | HMDB is offered to the public as a freely available resource. Use and re-distribution of the data, in whole or in part, for commercial purposes requires explicit permission of the authors and explicit acknowledgment of the source material (HMDB) and the original publication (see the HMDB citing page). We ask that users who download significant portions of the database cite the HMDB paper in any resulting publications. | |
Melting Point |
119 - 122 °C | |
Record name | D-Fructose | |
Source | Human Metabolome Database (HMDB) | |
URL | http://www.hmdb.ca/metabolites/HMDB0000660 | |
Description | The Human Metabolome Database (HMDB) is a freely available electronic database containing detailed information about small molecule metabolites found in the human body. | |
Explanation | HMDB is offered to the public as a freely available resource. Use and re-distribution of the data, in whole or in part, for commercial purposes requires explicit permission of the authors and explicit acknowledgment of the source material (HMDB) and the original publication (see the HMDB citing page). We ask that users who download significant portions of the database cite the HMDB paper in any resulting publications. | |
Retrosynthesis Analysis
AI-Powered Synthesis Planning: Our tool employs the Template_relevance Pistachio, Template_relevance Bkms_metabolic, Template_relevance Pistachio_ringbreaker, Template_relevance Reaxys, Template_relevance Reaxys_biocatalysis model, leveraging a vast database of chemical reactions to predict feasible synthetic routes.
One-Step Synthesis Focus: Specifically designed for one-step synthesis, it provides concise and direct routes for your target compounds, streamlining the synthesis process.
Accurate Predictions: Utilizing the extensive PISTACHIO, BKMS_METABOLIC, PISTACHIO_RINGBREAKER, REAXYS, REAXYS_BIOCATALYSIS database, our tool offers high-accuracy predictions, reflecting the latest in chemical research and data.
Strategy Settings
Precursor scoring | Relevance Heuristic |
---|---|
Min. plausibility | 0.01 |
Model | Template_relevance |
Template Set | Pistachio/Bkms_metabolic/Pistachio_ringbreaker/Reaxys/Reaxys_biocatalysis |
Top-N result to add to graph | 6 |
Feasible Synthetic Routes
Q & A
Q1: What is the molecular formula and weight of β-D-fructose?
A1: The molecular formula for β-D-fructose is C6H12O6, and its molecular weight is 180.16 g/mol.
Q2: How can you differentiate between α-D-fructofuranose and β-D-fructofuranose using NMR spectroscopy?
A2: NMR studies demonstrated that rat hepatic 6-phosphofructo-2-kinase exclusively produces β-D-fructose-2,6-bisphosphate from β-D-fructose-6-phosphate. A specific long-range coupling constant (4JH-3, P-2) of 1.06 Hz between P-2 and H-3, characteristic of the β-anomer, was observed. The absence of a similar coupling to the H-1a peak further confirmed the absence of the α-anomer. []
Q3: Does the molecular mobility of β-D-fructose in its amorphous solid state change with time?
A3: Yes, the molecular mobility of amorphous β-D-fructose is affected by physical aging. Studies using thermally stimulated depolarization currents (TSDC) have shown that the slower motional modes of the secondary relaxation in amorphous fructose exhibit a significant dependence on aging, indicating structural relaxation within the glass. []
Q4: How does β-D-fructose influence adenine aggregation, and what is the biological relevance?
A4: β-D-fructose exhibits a relatively weak cosolvent effect on adenine aggregation compared to other sugars like D-galactose. Molecular mechanics simulations suggest that this difference arises from the inability of β-D-fructose, with its two polar faces, to effectively engage in CH-pi stacking interactions with the aromatic structure of adenine. In contrast, β-D-galactopyranose, with its apolar surface, readily forms CH-pi stacking interactions, promoting adenine solubility. []
Q5: Can β-D-fructose form complexes with metal ions?
A5: Yes, research has demonstrated the formation of a stable complex between Zn(II) and β-D-fructose 2,6-bisphosphate. This complex activates enzymes like bis(5'-guanosyl)tetraphosphatase and dinucleoside triphosphatase, which can be subsequently inhibited by Zn(II). []
Q6: How does β-D-fructose influence ascorbate transport in biological systems?
A6: While β-D-fructose itself doesn't directly impact ascorbate transport, α-D-glucose and related analogues like 3-O-methyl-D-glucose competitively inhibit ascorbate transport in retinal capillary pericytes [] and retinal pigment epithelial cells []. This suggests a shared facilitated carrier diffusion system for ascorbate and specific sugar analogues. Notably, β-D-fructose does not show this inhibitory effect, indicating structural specificity for the transporter.
Q7: How is computational chemistry used to study β-D-fructose and its derivatives?
A8: Computational methods like density functional theory (DFT) are valuable tools in studying β-D-fructose. For example, DFT calculations were crucial in identifying the structure of radiation-induced radicals in β-D-fructose single crystals, providing insights into their hyperfine coupling tensors and molecular geometries. []
Q8: Have there been any studies exploring the structure-activity relationships (SAR) of β-D-fructose derivatives?
A9: Yes, research exploring nitrogen-in-the-ring analogs of β-D-fructose, specifically 2,5-dideoxy-2,5-imino-D-mannitol (a mimic of β-D-fructofuranose), revealed its potent inhibitory activity against mammalian β-galactosidases. This finding highlights the impact of structural modifications on the biological activity of sugar derivatives. []
Haftungsausschluss und Informationen zu In-Vitro-Forschungsprodukten
Bitte beachten Sie, dass alle Artikel und Produktinformationen, die auf BenchChem präsentiert werden, ausschließlich zu Informationszwecken bestimmt sind. Die auf BenchChem zum Kauf angebotenen Produkte sind speziell für In-vitro-Studien konzipiert, die außerhalb lebender Organismen durchgeführt werden. In-vitro-Studien, abgeleitet von dem lateinischen Begriff "in Glas", beinhalten Experimente, die in kontrollierten Laborumgebungen unter Verwendung von Zellen oder Geweben durchgeführt werden. Es ist wichtig zu beachten, dass diese Produkte nicht als Arzneimittel oder Medikamente eingestuft sind und keine Zulassung der FDA für die Vorbeugung, Behandlung oder Heilung von medizinischen Zuständen, Beschwerden oder Krankheiten erhalten haben. Wir müssen betonen, dass jede Form der körperlichen Einführung dieser Produkte in Menschen oder Tiere gesetzlich strikt untersagt ist. Es ist unerlässlich, sich an diese Richtlinien zu halten, um die Einhaltung rechtlicher und ethischer Standards in Forschung und Experiment zu gewährleisten.