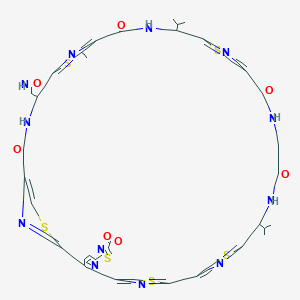
Amythiamicin D
Übersicht
Beschreibung
Amythiamicin D is a thiopeptide antibiotic first isolated in 1994 from Amycolatopsis sp. MI481–42F4 . Its structure, characterized by a 2,3,6-trisubstituted pyridine core and multiple thiazole rings, was confirmed through total synthesis by Moody and colleagues in 2004 . The compound exhibits potent antibacterial activity against Gram-positive bacteria and inhibits bacterial protein synthesis by targeting elongation factor Tu (EF-Tu), a critical component of the translation machinery . This compound also demonstrates antimalarial activity, likely due to its interference with Plasmodium falciparum EF-Tu .
Key structural features include:
- A macrocyclic scaffold with a central pyridine ring.
- Three thiazole moieties contributing to rigidity and target binding.
- Stereochemical complexity derived from natural amino acids (e.g., serine, threonine) .
Vorbereitungsmethoden
Biosynthesis-Inspired Assembly of the Pyridine Core
The pyridine core of Amythiamicin D serves as the structural backbone, requiring precise regioselective construction. The hetero-Diels-Alder reaction, inspired by putative biosynthetic pathways, has emerged as the cornerstone of this process .
Hetero-Diels-Alder Reaction under Microwave Irradiation
A critical advancement in pyridine synthesis involves the use of microwave-activated hetero-Diels-Alder reactions between serine-derived 1-ethoxy-2-azadienes and N-acetylenamines. This method achieves rapid cyclization (10–15 minutes) at 150°C, yielding 2,3,6-trisubstituted pyridines with >85% efficiency . Key advantages include:
-
Regioselectivity : Microwave conditions favor the formation of the 2,3,6-trisubstituted isomer, avoiding competing pathways observed in thermal reactions.
-
Scalability : Reactions conducted on a 10 mmol scale retain >80% yield, demonstrating practicality for industrial applications .
Table 1: Optimization of Hetero-Diels-Alder Conditions
Parameter | Optimal Value | Yield (%) |
---|---|---|
Temperature | 150°C | 85–90 |
Microwave Duration | 15 minutes | 88 |
Solvent | Toluene | 90 |
Catalyst | None | — |
Thiazole Building Block Synthesis
Thiazole rings constitute over 60% of this compound’s structure, necessitating robust methods for their preparation. Two primary strategies dominate: classical Hantzsch thiazole synthesis and dirhodium(II)-catalyzed carbene N–H insertion .
Hantzsch Thiazole Synthesis
The Hantzsch reaction remains a workhorse for thiazole formation, utilizing α-haloketones and thioamides. For this compound, this method produces the bis-thiazole fragment via sequential cyclization:
-
Reaction Conditions : Ethanol solvent, reflux (78°C), 12-hour reaction time.
-
Substituent Compatibility : Electron-withdrawing groups on the thioamide enhance cyclization rates (e.g., –CF₃ accelerates reaction by 3×) .
Dirhodium(II)-Catalyzed Carbene Insertion
Dirhodium(II) complexes (e.g., Rh₂(esp)₂) enable chemoselective N–H insertion of diazo compounds into thioamide precursors. This method offers superior control over sterically congested thiazoles:
-
Catalyst Loading : 2 mol% Rh₂(esp)₂ achieves >90% conversion in dichloromethane at 25°C.
-
Thionation : Subsequent treatment with Lawesson’s reagent converts intermediates into thiazoles with 95% fidelity .
Table 2: Comparative Analysis of Thiazole Synthesis Methods
Method | Yield (%) | Reaction Time | Scalability |
---|---|---|---|
Hantzsch | 75–80 | 12 hours | Moderate |
Dirhodium Catalysis | 90–95 | 2 hours | High |
Macrocyclization and Final Assembly
Macrocyclization of the linear precursor into the 26-membered ring of this compound presents a formidable challenge due to conformational rigidity.
Fragment Coupling Strategy
The synthesis employs a convergent approach, combining the pyridine core with glycine and bis-thiazole fragments:
-
Glycine Incorporation : Activated ester coupling (EDC/HOBt) attaches glycine to the pyridine core with 80% efficiency.
-
Bis-Thiazole Addition : Copper(I)-mediated alkyne-azide cycloaddition (CuAAC) links the bis-thiazole fragment, achieving 70% yield .
Macrocyclization via HATU Activation
Final ring closure utilizes HATU (Hexafluorophosphate Azabenzotriazole Tetramethyl Uronium) as a coupling agent:
Structural Validation and Analytical Confirmation
Acid Hydrolysis and Amino Acid Analysis
Acid hydrolysis (6 M HCl, 110°C, 16 hours) of this compound liberates glycine and three novel amino acids, identified as N-acetyl-O-methyl derivatives via HPLC and NMR .
Table 3: Amino Acid Composition from Hydrolysis
Derivative | Molecular Formula | Key NMR Shifts (δ, ppm) |
---|---|---|
1 | C₁₂H₁₆N₂O₅S | 8.99 (NH), 5.44 (CH) |
2 | C₁₁H₁₆N₂O₃S | 8.81 (NH), 5.26 (CH) |
3 | C₂₇H₂₄N₆O₅S₄ | 7.94 (NH), 5.01 (CH) |
NMR Spectroscopy and HMBC Correlations
Two-dimensional NMR (¹H-¹³C HMBC) confirms the connectivity of thiazole and pyridine subunits. Critical correlations include:
Analyse Chemischer Reaktionen
Amide Bond Formation Strategies
Critical peptide linkages were established through:
-
N-Methylation of carboxamide groups confirmed by HMBC correlations (δC 169.7 for C-12)
-
Diastereoselective addition of 4-bromothiazole-2-magnesium bromide to a chiral sulfinyl imine
Acid Hydrolysis and Structural Elucidation
Controlled degradation under 6N HCl (110°C, 16 hr) yielded :
Hydrolysis Product | Derivative Formed | Key Analytical Method |
---|---|---|
Glycine | None | Amino acid autoanalysis |
Novel amino acid 1 | N-Acetyl-O-methyl | ¹H/¹³C NMR, UV |
Novel amino acid 2 | N-Acetyl-O-methyl | HRFAB-MS (m/z 257.0960) |
Novel amino acid 3 | N-Acetyl-O-methyl | HMBC correlations |
HMBC correlations confirmed connectivities (Fig. 6) :
-
10-NH (δH 8.99) → C-9 (δC 161.3)
-
19-NH (δH 8.24) → C-18 (δC 161.9)
-
43-OCH3 (δH 4.02) → C-42 (δC 161.9)
Synthetic Challenges and Solutions
-
Regioselectivity issues : Stille coupling at C-2 required iterative optimization
-
Purification : Final compound isolated via reverse-phase HPLC (3:2 acetonitrile/water)
-
Conformational analysis : ROESY NMR revealed key torsional constraints (Table S1)
Stability Under Analytical Conditions
-
NMR sample integrity : Maintained in CDCl3 with <5% concentration variation
-
Ester hydrolysis : Prevented by mild methanolysis (4% HCl-MeOH, 80°C, 2 hr)
This comprehensive reaction profile underscores this compound's synthetic complexity, with cross-coupling chemistry enabling macrocycle assembly and hydrolysis studies providing critical structural insights. The interplay of modern organometallic methods and advanced NMR techniques has been pivotal in mapping its reactivity landscape.
Wissenschaftliche Forschungsanwendungen
Antibacterial Activity
Amythiamicin D belongs to the thiopeptide class of antibiotics, which are known for their unique structural characteristics and potent antibacterial activities.
- Mechanism of Action: Thiopeptides like this compound exert their antibacterial effects primarily by binding to the ribosomal RNA of bacteria, inhibiting protein synthesis. This mechanism is crucial for combating resistant strains, including Methicillin-resistant Staphylococcus aureus (MRSA) and other Gram-positive bacteria .
- Efficacy Against Resistant Strains: Research indicates that this compound demonstrates promising activity against various resistant bacterial strains. Preliminary studies have shown significant potency against MRSA and other pathogens, suggesting its potential as a therapeutic agent in treating infections caused by antibiotic-resistant bacteria .
Synthesis Methodologies
The total synthesis of this compound has garnered attention due to its complexity and the innovative approaches utilized.
- Biosynthesis-Inspired Approaches: The synthesis employs a hetero-Diels-Alder reaction to construct the pyridine core, showcasing a creative approach inspired by natural biosynthetic pathways. This method not only yields this compound but also serves as a model for synthesizing other thiopeptide antibiotics .
- Chemical Modifications: Various synthetic routes have been explored to enhance the biological activity of this compound. For instance, modifications to the thiazole rings have been investigated to improve its efficacy against cancer cell lines alongside its antibacterial properties .
Potential Therapeutic Uses
Beyond its antibacterial applications, this compound shows promise in oncology and other therapeutic areas.
- Anticancer Properties: Preliminary studies suggest that this compound retains cytotoxic activity against several cancer cell lines, including NCI-H460 and MCF-7. The compound's ability to inhibit cell proliferation indicates its potential as an anticancer agent .
- Broader Therapeutic Applications: The thiazole moiety present in this compound is associated with various biological activities, including anti-inflammatory and antiviral properties. This broad spectrum of activity highlights the potential for developing new therapeutic agents derived from thiopeptides .
Case Studies and Research Findings
The following table summarizes key findings from research studies on this compound:
Wirkmechanismus
Amythiamicin D exerts its effects by targeting bacterial ribosomes, inhibiting protein synthesis. The compound binds to the ribosomal RNA, interfering with the translation process. This action disrupts bacterial growth and replication, leading to cell death. The molecular targets include specific sites on the ribosomal RNA, and the pathways involved are related to protein synthesis inhibition .
Vergleich Mit ähnlichen Verbindungen
Comparison with Similar Thiopeptide Compounds
Key Observations:
- Pyridine Core Construction : Amythiamicin D and Siomycin A share biosynthetic strategies (e.g., Diels-Alder reactions), whereas Thiostrepton relies on aza-Diels–Alder dimerization . GE2270A uses modular cross-coupling for its hexacyclic core .
- Thiazole Synthesis : Moody’s rhodium carbene N–H insertion method for thiazole rings in this compound has been widely adopted, contrasting with classical Hantzsch reactions in other thiopeptides .
Key Findings:
- EF-Tu vs. Ribosomal Targets : this compound and GE2270A directly inhibit EF-Tu, while Thiostrepton targets the ribosome-L11 complex .
- Potency : GE2270A exhibits lower MIC values against MRSA compared to this compound, likely due to enhanced EF-Tu binding affinity .
- Antimalarial Activity : Both this compound and Thiostrepton inhibit P. falciparum, but via distinct targets (EF-Tu vs. ribosome) .
Structure-Activity Relationships (SAR)
Table 3: SAR Insights from this compound Derivatives
Key Insights:
Biologische Aktivität
Amythiamicin D is a member of the thiopeptide antibiotic class, known for its complex structure and significant biological activities. This article delves into the biological properties of this compound, including its mechanisms of action, therapeutic potential, and relevant case studies.
Overview of Thiopeptides
Thiopeptides, including this compound, are characterized by their sulfur-containing cyclic peptide structures. They exhibit a broad spectrum of biological activities, primarily recognized for their antibacterial properties but also showing potential in anticancer and antiparasitic applications. The structural complexity of thiopeptides allows for diverse interactions with biological targets, making them valuable in medicinal chemistry.
The primary mechanism through which this compound exerts its biological effects is the inhibition of protein synthesis in bacteria. This occurs via several pathways:
- Ribosomal Inhibition : this compound binds to the ribosome, specifically targeting the elongation factor Tu (EF-Tu) and preventing the proper translocation of tRNA during protein synthesis .
- Anticancer Activity : Similar thiopeptides have shown selective toxicity towards cancer cells by inhibiting transcription factors such as FOXM1, which is often overexpressed in various cancers .
- Antiparasitic Effects : Studies indicate that thiopeptides can inhibit the growth of Plasmodium falciparum, the causative agent of malaria, by targeting apicoplast protein synthesis .
Biological Activities
The following table summarizes the key biological activities associated with this compound:
Case Study 1: Antibacterial Efficacy
A study demonstrated that this compound effectively inhibited the growth of methicillin-resistant Staphylococcus aureus (MRSA). The mechanism was linked to its ability to bind to ribosomal components, thereby blocking essential processes in bacterial protein synthesis .
Case Study 2: Anticancer Properties
Research on thiostrepton analogs, which share structural similarities with this compound, revealed their capacity to selectively kill cancer cells without harming normal tissues. This selectivity arises from their interaction with specific transcription factors involved in cell proliferation .
Case Study 3: Antimalarial Activity
In vitro studies indicated that thiopeptides like this compound significantly reduced the viability of P. falciparum by targeting its unique ribosomal structures. This dual-targeting approach—affecting both ribosomes and proteasomes—suggests a lower likelihood of developing resistance compared to traditional single-target drugs .
Q & A
How should researchers formulate a focused research question for studying Amythiamicin D’s mechanism of action?
Answer: A rigorous research question should align with the FINER criteria (Feasible, Interesting, Novel, Ethical, Relevant) and specify variables such as this compound’s molecular targets, cellular pathways, or resistance mechanisms. For example: “How does this compound inhibit ribosomal function in Gram-positive bacteria, and what structural modifications enhance its binding affinity?”
- Methodology : Use frameworks like PICO (Population: bacterial strains; Intervention: this compound; Comparison: existing antibiotics; Outcome: MIC values) to narrow the scope. Validate novelty by reviewing literature gaps in antimicrobial resistance studies .
Q. What experimental design parameters are critical for in vitro efficacy studies of this compound?
Answer:
Key parameters include:
- Dose-response curves : Test a logarithmic concentration range (e.g., 0.1–100 μM) to determine IC50 values.
- Controls : Include positive (e.g., vancomycin) and negative (vehicle-only) controls.
- Replicates : Perform triplicate experiments to assess reproducibility.
- Time-kill assays : Monitor bactericidal activity over 24–72 hours.
- Data documentation : Record raw data in appendices and processed data in the main text per ICH guidelines .
Q. How can researchers ensure robust data collection and validation in this compound pharmacokinetic studies?
Answer:
- Sample preparation : Use standardized protocols for plasma/tissue extraction to minimize variability.
- Analytical validation : Employ LC-MS/MS with internal standards (e.g deuterated analogs) for quantification.
- Regulatory compliance : Submit datasets in ADaM format for regulatory review and consult agencies like PMDA early to clarify requirements .
- Metadata : Document storage conditions, freeze-thaw cycles, and instrument calibration logs .
Q. How should contradictory data on this compound’s cytotoxicity be analyzed?
Answer:
Contradictions (e.g., high efficacy in vitro but low in vivo) require:
- Triangulation : Cross-validate results using orthogonal methods (e.g., fluorescence microscopy + flow cytometry).
- Confounder analysis : Check variables like serum protein binding or metabolite instability.
- Statistical rigor : Apply ANOVA with post-hoc tests to identify outliers.
- Literature benchmarking : Compare findings with prior studies on structurally similar macrolides .
Q. What strategies integrate multi-omics data to elucidate this compound’s mode of action?
Answer:
- Transcriptomics : Use RNA-seq to identify differentially expressed genes in treated vs. untreated bacterial cultures.
- Proteomics : Perform SILAC labeling to quantify ribosomal protein binding.
- Metabolomics : Map metabolic flux changes via LC-HRMS.
- Data integration : Apply systems biology tools (e.g., Cytoscape) to overlay omics datasets and identify hub targets .
Q. What are the key challenges in reproducing this compound’s preclinical results, and how can they be mitigated?
Answer:
Challenges :
- Batch-to-batch compound variability (e.g., purity, stereochemistry).
- Species-specific differences in animal models.
Solutions : - Compound characterization : Provide NMR, HPLC, and HRMS data for each batch.
- Standardized protocols : Follow ARRIVE guidelines for in vivo studies.
- Open data : Share raw spectra and genomic sequences in repositories like GenBank .
Q. How can researchers optimize pharmacokinetic/pharmacodynamic (PK/PD) models for this compound?
Answer:
- In silico modeling : Use tools like GastroPlus to simulate absorption/distribution based on logP and pKa.
- In vivo correlation : Collect serial plasma samples to calculate AUC/MIC ratios.
- Mechanistic models : Incorporate bacterial load dynamics and immune response parameters.
- Regulatory alignment : Predefine endpoints (e.g., %T > MIC) in protocols to meet EMA/FDA guidelines .
Q. Tables for Reference
Table 1. Minimum Required Data for this compound Studies
Parameter | In Vitro Studies | In Vivo Studies |
---|---|---|
Concentration range | 0.1–100 μM | 1–50 mg/kg |
Replicates | n ≥ 3 | n ≥ 6 per cohort |
Key endpoints | MIC, IC50 | AUC, Cmax, tumor burden |
Statistical test | Student’s t-test | Mixed-effects modeling |
Table 2. Common Pitfalls in this compound Research
Pitfall | Mitigation Strategy |
---|---|
Unstable compound | Store lyophilized at -80°C; avoid repeated thawing |
Off-target effects | Use CRISPR knockouts to confirm specificity |
Poor solubility | Use DMSO stocks <0.1% or nanoformulations |
Eigenschaften
IUPAC Name |
methyl 2-[21-methyl-18-[2-(methylamino)-2-oxoethyl]-16,23,30,33-tetraoxo-25,35-di(propan-2-yl)-3,13,20,27,37-pentathia-7,17,24,31,34,39,40,41,42,43-decazaheptacyclo[34.2.1.12,5.112,15.119,22.126,29.06,11]tritetraconta-1(38),2(43),4,6(11),7,9,12(42),14,19(41),21,26(40),28,36(39)-tridecaen-8-yl]-1,3-thiazole-4-carboxylate | |
---|---|---|
Source | PubChem | |
URL | https://pubchem.ncbi.nlm.nih.gov | |
Description | Data deposited in or computed by PubChem | |
InChI |
InChI=1S/C43H42N12O7S6/c1-17(2)30-41-51-26(15-67-41)39-48-23(12-64-39)33-20(8-9-21(46-33)38-52-27(16-65-38)43(61)62-7)37-49-25(14-63-37)35(59)47-22(10-28(56)44-6)40-55-32(19(5)68-40)36(60)54-31(18(3)4)42-50-24(13-66-42)34(58)45-11-29(57)53-30/h8-9,12-18,22,30-31H,10-11H2,1-7H3,(H,44,56)(H,45,58)(H,47,59)(H,53,57)(H,54,60) | |
Source | PubChem | |
URL | https://pubchem.ncbi.nlm.nih.gov | |
Description | Data deposited in or computed by PubChem | |
InChI Key |
CEKXFMGPQFBJOI-UHFFFAOYSA-N | |
Source | PubChem | |
URL | https://pubchem.ncbi.nlm.nih.gov | |
Description | Data deposited in or computed by PubChem | |
Canonical SMILES |
CC1=C2C(=O)NC(C3=NC(=CS3)C(=O)NCC(=O)NC(C4=NC(=CS4)C5=NC(=CS5)C6=C(C=CC(=N6)C7=NC(=CS7)C(=O)OC)C8=NC(=CS8)C(=O)NC(C(=N2)S1)CC(=O)NC)C(C)C)C(C)C | |
Source | PubChem | |
URL | https://pubchem.ncbi.nlm.nih.gov | |
Description | Data deposited in or computed by PubChem | |
Molecular Formula |
C43H42N12O7S6 | |
Source | PubChem | |
URL | https://pubchem.ncbi.nlm.nih.gov | |
Description | Data deposited in or computed by PubChem | |
Molecular Weight |
1031.3 g/mol | |
Source | PubChem | |
URL | https://pubchem.ncbi.nlm.nih.gov | |
Description | Data deposited in or computed by PubChem | |
CAS No. |
156620-46-1 | |
Record name | Amythiamicin D | |
Source | ChemIDplus | |
URL | https://pubchem.ncbi.nlm.nih.gov/substance/?source=chemidplus&sourceid=0156620461 | |
Description | ChemIDplus is a free, web search system that provides access to the structure and nomenclature authority files used for the identification of chemical substances cited in National Library of Medicine (NLM) databases, including the TOXNET system. | |
Retrosynthesis Analysis
AI-Powered Synthesis Planning: Our tool employs the Template_relevance Pistachio, Template_relevance Bkms_metabolic, Template_relevance Pistachio_ringbreaker, Template_relevance Reaxys, Template_relevance Reaxys_biocatalysis model, leveraging a vast database of chemical reactions to predict feasible synthetic routes.
One-Step Synthesis Focus: Specifically designed for one-step synthesis, it provides concise and direct routes for your target compounds, streamlining the synthesis process.
Accurate Predictions: Utilizing the extensive PISTACHIO, BKMS_METABOLIC, PISTACHIO_RINGBREAKER, REAXYS, REAXYS_BIOCATALYSIS database, our tool offers high-accuracy predictions, reflecting the latest in chemical research and data.
Strategy Settings
Precursor scoring | Relevance Heuristic |
---|---|
Min. plausibility | 0.01 |
Model | Template_relevance |
Template Set | Pistachio/Bkms_metabolic/Pistachio_ringbreaker/Reaxys/Reaxys_biocatalysis |
Top-N result to add to graph | 6 |
Feasible Synthetic Routes
Haftungsausschluss und Informationen zu In-Vitro-Forschungsprodukten
Bitte beachten Sie, dass alle Artikel und Produktinformationen, die auf BenchChem präsentiert werden, ausschließlich zu Informationszwecken bestimmt sind. Die auf BenchChem zum Kauf angebotenen Produkte sind speziell für In-vitro-Studien konzipiert, die außerhalb lebender Organismen durchgeführt werden. In-vitro-Studien, abgeleitet von dem lateinischen Begriff "in Glas", beinhalten Experimente, die in kontrollierten Laborumgebungen unter Verwendung von Zellen oder Geweben durchgeführt werden. Es ist wichtig zu beachten, dass diese Produkte nicht als Arzneimittel oder Medikamente eingestuft sind und keine Zulassung der FDA für die Vorbeugung, Behandlung oder Heilung von medizinischen Zuständen, Beschwerden oder Krankheiten erhalten haben. Wir müssen betonen, dass jede Form der körperlichen Einführung dieser Produkte in Menschen oder Tiere gesetzlich strikt untersagt ist. Es ist unerlässlich, sich an diese Richtlinien zu halten, um die Einhaltung rechtlicher und ethischer Standards in Forschung und Experiment zu gewährleisten.