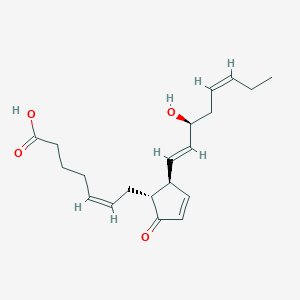
Prostaglandin A3
Übersicht
Beschreibung
Prostaglandin A3 is a member of the prostaglandin family, which are lipid compounds derived from fatty acids. These compounds play crucial roles in various physiological processes, including inflammation, blood flow, and the formation of blood clots. This compound is particularly notable for its involvement in regulating inflammation and immune responses.
Vorbereitungsmethoden
Synthesewege und Reaktionsbedingungen: Die Synthese von Prostaglandin A3 beinhaltet typischerweise die Verwendung von Lactonen als Schlüsselzwischenprodukte. Die Corey-Lacton-Methode ist eine der effizientesten und am häufigsten verwendeten Synthesewege. Diese Methode beinhaltet die Bildung von δ-Lactonen und γ-Lactonen, die dann durch eine Reihe von stereogesteuerten Reaktionen in das gewünschte Prostaglandin umgewandelt werden .
Industrielle Produktionsverfahren: Die industrielle Produktion von this compound verwendet oft chemoenzymatische Synthesemethoden. Diese Methoden kombinieren chemische und enzymatische Schritte, um hohe Ausbeuten und Reinheiten zu erreichen. Der Prozess beginnt in der Regel mit der enzymatischen Umwandlung von Arachidonsäure in Prostaglandin-Zwischenprodukte, gefolgt von chemischen Modifikationen, um das Endprodukt zu erhalten .
Analyse Chemischer Reaktionen
1.1. Lactonization Strategies
PGA₃ is synthesized via δ-lactone or γ-lactone intermediates in multi-step sequences (Figure 1):
Key Observations :
-
The Corey-Nicolaou method requires protecting hydroxyl groups (e.g., THP ethers) to prevent side reactions .
-
Metal-catalyzed routes enable enantioselective synthesis via chiral allylic alcohol intermediates .
1.2. Allene Oxide Biosynthetic Pathway
PGA₃ forms via cyclization of 8-hydroxy-15S-hydroperoxy eicosanoids in the presence of allene oxide synthase (Table 2):
Mechanism :
-
Lipoxygenase oxidizes arachidonic acid (AA) or eicosapentaenoic acid (EPA) to hydroperoxides.
-
Allene oxide synthase dehydrates hydroperoxides to unstable allene oxides.
2.1. Enzymatic Conversion
PGA₃ serves as a precursor for bioactive metabolites:
Key Finding : TXA₃ derived from PGA₃ shows 10-fold weaker platelet aggregation compared to TXA₂ .
3.1. pH-Dependent Degradation
PGA₃ undergoes retro-aldol cleavage under basic conditions (Table 3):
pH | Temperature (°C) | Half-Life (h) | Degradation Product | Source |
---|---|---|---|---|
7.4 | 37 | 48 | 15-keto-PGA₃ | |
9.0 | 25 | 2.5 | 9,15-Diketo-prostanoate |
Implication : Stability in biological systems (pH 7.4) allows PGA₃ to exert prolonged effects compared to acidic prostaglandins .
4.1. Receptor Binding
PGA₃ interacts with prostaglandin transporters (PGT) and receptors (Table 4):
Target | Affinity (Kd, nM) | Effect | Source |
---|---|---|---|
EP3 Receptor | 320 ± 45 | Inhibition of cAMP synthesis | |
PPARγ | 110 ± 20 | Anti-inflammatory gene activation |
Notable Activity : PPARγ activation by PGA₃ reduces NF-κB-driven inflammation .
5.1. Halogenation
δ-Lactone intermediates enable 9β-halogenation (Table 5):
Reagent | Product | Yield (%) | Application | Source |
---|---|---|---|---|
N-Bromosuccinimide | 9β-Bromo-PGA₃ | 68 | Anticancer lead compound | |
Cl₂/CCl₄ | 9β-Chloro-PGA₃ | 72 | Cyclopentane analog synthesis |
Rationale : Halogenation enhances electrophilicity, improving binding to cellular targets .
Wissenschaftliche Forschungsanwendungen
Role in Insect Development
Recent studies have highlighted the impact of PGA3 on insect development, particularly in termites. Research conducted on Odontotermes formosanus indicates that PGA3 regulates colony development by affecting the worker-to-soldier ratio (WSR).
- Key Findings:
- Under specific nutritional conditions (MGL nutrition), the synthesis of PGA3 significantly increased, leading to a reduced WSR in colonies.
- The study utilized LC–MS/MS and ICP-MS analyses to confirm elevated levels of PGA3 and calcium concentrations in the branches consumed by termites.
- Increased peroxidase (POD) activity was also observed, suggesting a biochemical pathway involving PGA3 that inhibits worker development .
This research introduces potential environmentally friendly biological control methods for termite populations by manipulating PGA3 levels.
Implications in Reproductive Biology
PGA3 has been studied for its interactions with sperm and seminal plasma proteins, particularly in bovines. Evidence suggests that proteins such as BSP A3 and prostaglandin D-synthase (PGDS) interact with sperm during ejaculation.
- Key Findings:
These findings are crucial for understanding fertility mechanisms and developing strategies to enhance reproductive efficiency in livestock.
Environmental Implications
The modulation of insect behavior and development through PGA3 presents opportunities for ecological management. By understanding how PGA3 influences insect physiology, researchers can develop biocontrol agents that target pest species without harming beneficial organisms.
Wirkmechanismus
Prostaglandin A3 exerts its effects by binding to specific receptors on the surface of target cells. This binding activates intracellular signaling pathways, leading to various physiological responses. The primary molecular targets of this compound include receptors involved in inflammation and immune regulation. The activation of these receptors triggers a cascade of events that modulate the activity of enzymes, transcription factors, and other proteins involved in these processes .
Vergleich Mit ähnlichen Verbindungen
Prostaglandin A3 ist Teil einer größeren Familie von Verbindungen, die als Eicosanoide bekannt sind, zu denen auch Thromboxane und Leukotriene gehören. Diese Verbindungen teilen einen gemeinsamen biosynthetischen Weg, unterscheiden sich aber in ihren Strukturen und Funktionen. Zum Beispiel:
Prostaglandin E2: Bekannt für seine Rolle bei der Induktion von Fieber und Schmerzen.
Prostaglandin F2α: Beteiligt an der Kontraktion der glatten Muskulatur und an Fortpflanzungsprozessen.
Thromboxan A2: Fördert die Thrombozytenaggregation und Vasokonstriktion.
Leukotrien B4: Beteiligt an der Entzündungsreaktion und Chemotaxis von Immunzellen.
This compound ist einzigartig in seiner spezifischen Rolle bei der Modulation von Entzündungen und Immunreaktionen, was es zu einer wertvollen Verbindung für die Forschung und therapeutische Anwendungen macht .
Biologische Aktivität
Prostaglandin A3 (PGA3) is a biologically active lipid mediator derived from eicosapentaenoic acid (EPA), a polyunsaturated fatty acid found in fish oils. PGA3 is part of the prostaglandin family, which plays critical roles in various physiological processes, including inflammation, immune response, and cellular signaling. This article explores the biological activity of PGA3, highlighting its mechanisms of action, effects on different biological systems, and implications for health and disease.
PGA3 exerts its biological effects primarily through its interaction with specific prostaglandin receptors, notably the EP2 and EP4 receptors. These G protein-coupled receptors are involved in mediating various cellular responses, including vasodilation, inhibition of platelet aggregation, and modulation of inflammatory processes . The binding affinity of PGA3 for these receptors suggests that it may play a significant role in cardiovascular health and inflammation regulation.
Biological Effects
-
Anti-Inflammatory Properties :
- PGA3 has been shown to inhibit the production of pro-inflammatory cytokines and chemokines. This effect is particularly relevant in conditions such as arthritis and other inflammatory diseases .
- In a study involving human neutrophils, PGA3 was demonstrated to reduce the migration of these cells towards inflammatory stimuli, indicating its potential as an anti-inflammatory agent .
-
Regulation of Immune Responses :
- Research indicates that PGA3 can modulate immune cell activity. For instance, it influences the differentiation and function of T cells and macrophages, promoting an anti-inflammatory phenotype .
- In insect models, increased levels of PGA3 were associated with altered colony development in termites, suggesting a role in regulating social behavior and development through immune modulation .
- Effects on Vascular Function :
Case Study 1: this compound in Inflammation
A recent study examined the effects of PGA3 on human neutrophils exposed to inflammatory stimuli. The results showed that treatment with PGA3 significantly reduced neutrophil migration across epithelial barriers, underscoring its potential as a therapeutic agent in inflammatory diseases such as asthma and chronic obstructive pulmonary disease (COPD) .
Case Study 2: this compound and Termite Development
Another study focused on the role of PGA3 in regulating the colony development of Odontotermes formosanus (a species of termite). The findings indicated that increased levels of PGA3 due to specific nutritional interventions led to a lower worker-to-soldier ratio in colonies, suggesting that PGA3 may influence social structure and development through immune-related pathways .
Data Tables
Biological Activity | Effect | Mechanism |
---|---|---|
Anti-inflammatory | Inhibition of cytokine production | Receptor-mediated signaling |
Immune modulation | Altered T cell differentiation | Interaction with immune cell receptors |
Vascular function | Vasodilation | Activation of EP2/EP4 receptors |
Colony development | Reduced worker-to-soldier ratio | Regulation of immune responses in insects |
Eigenschaften
IUPAC Name |
(Z)-7-[(1R,2S)-2-[(1E,3S,5Z)-3-hydroxyocta-1,5-dienyl]-5-oxocyclopent-3-en-1-yl]hept-5-enoic acid | |
---|---|---|
Source | PubChem | |
URL | https://pubchem.ncbi.nlm.nih.gov | |
Description | Data deposited in or computed by PubChem | |
InChI |
InChI=1S/C20H28O4/c1-2-3-6-9-17(21)14-12-16-13-15-19(22)18(16)10-7-4-5-8-11-20(23)24/h3-4,6-7,12-18,21H,2,5,8-11H2,1H3,(H,23,24)/b6-3-,7-4-,14-12+/t16-,17-,18+/m0/s1 | |
Source | PubChem | |
URL | https://pubchem.ncbi.nlm.nih.gov | |
Description | Data deposited in or computed by PubChem | |
InChI Key |
KSIRMUMXJFWKAC-FHJHOUOTSA-N | |
Source | PubChem | |
URL | https://pubchem.ncbi.nlm.nih.gov | |
Description | Data deposited in or computed by PubChem | |
Canonical SMILES |
CCC=CCC(C=CC1C=CC(=O)C1CC=CCCCC(=O)O)O | |
Source | PubChem | |
URL | https://pubchem.ncbi.nlm.nih.gov | |
Description | Data deposited in or computed by PubChem | |
Isomeric SMILES |
CC/C=C\C[C@@H](/C=C/[C@H]1C=CC(=O)[C@@H]1C/C=C\CCCC(=O)O)O | |
Source | PubChem | |
URL | https://pubchem.ncbi.nlm.nih.gov | |
Description | Data deposited in or computed by PubChem | |
Molecular Formula |
C20H28O4 | |
Source | PubChem | |
URL | https://pubchem.ncbi.nlm.nih.gov | |
Description | Data deposited in or computed by PubChem | |
Molecular Weight |
332.4 g/mol | |
Source | PubChem | |
URL | https://pubchem.ncbi.nlm.nih.gov | |
Description | Data deposited in or computed by PubChem | |
Retrosynthesis Analysis
AI-Powered Synthesis Planning: Our tool employs the Template_relevance Pistachio, Template_relevance Bkms_metabolic, Template_relevance Pistachio_ringbreaker, Template_relevance Reaxys, Template_relevance Reaxys_biocatalysis model, leveraging a vast database of chemical reactions to predict feasible synthetic routes.
One-Step Synthesis Focus: Specifically designed for one-step synthesis, it provides concise and direct routes for your target compounds, streamlining the synthesis process.
Accurate Predictions: Utilizing the extensive PISTACHIO, BKMS_METABOLIC, PISTACHIO_RINGBREAKER, REAXYS, REAXYS_BIOCATALYSIS database, our tool offers high-accuracy predictions, reflecting the latest in chemical research and data.
Strategy Settings
Precursor scoring | Relevance Heuristic |
---|---|
Min. plausibility | 0.01 |
Model | Template_relevance |
Template Set | Pistachio/Bkms_metabolic/Pistachio_ringbreaker/Reaxys/Reaxys_biocatalysis |
Top-N result to add to graph | 6 |
Feasible Synthetic Routes
Haftungsausschluss und Informationen zu In-Vitro-Forschungsprodukten
Bitte beachten Sie, dass alle Artikel und Produktinformationen, die auf BenchChem präsentiert werden, ausschließlich zu Informationszwecken bestimmt sind. Die auf BenchChem zum Kauf angebotenen Produkte sind speziell für In-vitro-Studien konzipiert, die außerhalb lebender Organismen durchgeführt werden. In-vitro-Studien, abgeleitet von dem lateinischen Begriff "in Glas", beinhalten Experimente, die in kontrollierten Laborumgebungen unter Verwendung von Zellen oder Geweben durchgeführt werden. Es ist wichtig zu beachten, dass diese Produkte nicht als Arzneimittel oder Medikamente eingestuft sind und keine Zulassung der FDA für die Vorbeugung, Behandlung oder Heilung von medizinischen Zuständen, Beschwerden oder Krankheiten erhalten haben. Wir müssen betonen, dass jede Form der körperlichen Einführung dieser Produkte in Menschen oder Tiere gesetzlich strikt untersagt ist. Es ist unerlässlich, sich an diese Richtlinien zu halten, um die Einhaltung rechtlicher und ethischer Standards in Forschung und Experiment zu gewährleisten.