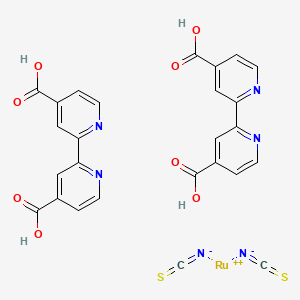
cis-Bis(isothiocyanato)bis(2,2'-bipyridyl-4,4'-dicarboxylato)ruthenium(II)
Übersicht
Beschreibung
cis-Bis(isothiocyanato)bis(2,2’-bipyridyl-4,4’-dicarboxylato)ruthenium(II): This compound is renowned for its application in dye-sensitized solar cells (DSSCs), where it acts as a sensitizer, facilitating the conversion of sunlight into electrical energy . The compound’s structure includes two bipyridyl ligands with carboxylate groups and two isothiocyanate ligands coordinated to a central ruthenium ion, which contributes to its unique photophysical properties .
Vorbereitungsmethoden
Synthetic Routes and Reaction Conditions: The synthesis of cis-Bis(isothiocyanato)bis(2,2’-bipyridyl-4,4’-dicarboxylato)ruthenium(II) typically involves the reaction of ruthenium trichloride with 2,2’-bipyridyl-4,4’-dicarboxylic acid in the presence of a base, followed by the addition of ammonium thiocyanate . The reaction is carried out under reflux conditions, and the product is purified through recrystallization or chromatography.
Industrial Production Methods: On an industrial scale, the production of this compound involves similar synthetic routes but optimized for large-scale operations. This includes the use of continuous flow reactors to ensure consistent quality and yield. The process is designed to minimize waste and improve the efficiency of the reaction .
Analyse Chemischer Reaktionen
Types of Reactions: cis-Bis(isothiocyanato)bis(2,2’-bipyridyl-4,4’-dicarboxylato)ruthenium(II) undergoes various chemical reactions, including:
Oxidation and Reduction: The compound can participate in redox reactions, where the ruthenium center can be oxidized or reduced, altering its oxidation state.
Substitution Reactions: The isothiocyanate ligands can be substituted with other ligands under appropriate conditions.
Common Reagents and Conditions:
Oxidation: Common oxidizing agents include cerium(IV) ammonium nitrate and potassium permanganate.
Reduction: Reducing agents such as sodium borohydride and hydrazine are often used.
Substitution: Ligand exchange reactions typically involve the use of coordinating solvents or other ligands like phosphines.
Major Products: The major products of these reactions depend on the specific conditions and reagents used. For example, oxidation may yield higher oxidation state ruthenium complexes, while substitution reactions can produce a variety of ruthenium complexes with different ligands .
Wissenschaftliche Forschungsanwendungen
Dye-Sensitized Solar Cells (DSSCs)
One of the primary applications of cis-bis(isothiocyanato)bis(2,2'-bipyridyl-4,4'-dicarboxylato)ruthenium(II) is in dye-sensitized solar cells. The compound serves as a sensitizer that absorbs sunlight and converts it into electrical energy through a series of photochemical reactions.
Photophysical Properties
- Absorption Spectrum : The N3 dye exhibits strong absorption in the visible region, particularly around 500-600 nm, making it effective for harnessing solar energy.
- Quantum Efficiency : The dye's ability to generate charge carriers upon light absorption contributes to high quantum efficiency in DSSCs.
Performance Metrics
Parameter | Value |
---|---|
Maximum Absorbance | ~550 nm |
Photovoltaic Conversion Efficiency | Up to 11% |
Stability | High under operational conditions |
Photochemical Applications
Beyond solar energy conversion, cis-bis(isothiocyanato)bis(2,2'-bipyridyl-4,4'-dicarboxylato)ruthenium(II) has shown potential in various photochemical applications:
Photocatalysis
The compound can act as a photocatalyst in organic synthesis and environmental remediation. Its ability to generate reactive oxygen species (ROS) upon light excitation allows it to facilitate reactions such as:
- Degradation of organic pollutants.
- Synthesis of fine chemicals through photoredox reactions.
Biological Applications
Recent studies have explored the biological implications of ruthenium complexes like N3 dye. Their properties can be leveraged for:
Anticancer Activity
Research indicates that cis-bis(isothiocyanato)bis(2,2'-bipyridyl-4,4'-dicarboxylato)ruthenium(II) may exhibit cytotoxic effects against certain cancer cell lines. The mechanism involves:
- Induction of apoptosis in malignant cells.
- Targeting cellular pathways involved in proliferation and survival.
Future Prospects
The versatility of cis-bis(isothiocyanato)bis(2,2'-bipyridyl-4,4'-dicarboxylato)ruthenium(II) paves the way for future research and applications:
- Hybrid Systems : Combining with other materials to improve efficiency in DSSCs.
- Bioconjugation : Exploring its use in targeted drug delivery systems where the compound could be conjugated with biomolecules for enhanced specificity.
Wirkmechanismus
The mechanism by which cis-Bis(isothiocyanato)bis(2,2’-bipyridyl-4,4’-dicarboxylato)ruthenium(II) exerts its effects involves the absorption of light, leading to the excitation of electrons from the ground state to an excited state . This excited state can then participate in electron transfer processes, either donating electrons to an acceptor or accepting electrons from a donor . In DSSCs, the excited electrons are injected into the conduction band of a semiconductor, typically titanium dioxide, generating an electric current .
Vergleich Mit ähnlichen Verbindungen
cis-Bis(isothiocyanato)(2,2’-bipyridyl-4,4’-dicarboxylato)(4,4’-di-nonyl-2’-bipyridyl)ruthenium(II) (Z-907): This compound is similar in structure but includes a hydrophobic bipyridyl ligand, which enhances its stability and efficiency in DSSCs.
Di-tetrabutylammonium cis-bis(isothiocyanato)bis(2,2’-bipyridyl-4,4’-dicarboxylato)ruthenium(II) (N719): This hydrophilic analogue is widely used in DSSCs for its high efficiency and stability.
Uniqueness: cis-Bis(isothiocyanato)bis(2,2’-bipyridyl-4,4’-dicarboxylato)ruthenium(II) is unique due to its balanced hydrophilic and hydrophobic properties, making it versatile for various applications. Its ability to efficiently convert sunlight into electricity while maintaining stability under different conditions sets it apart from other similar compounds .
Biologische Aktivität
cis-Bis(isothiocyanato)bis(2,2'-bipyridyl-4,4'-dicarboxylato)ruthenium(II), commonly referred to as N3 dye, is a ruthenium-based complex widely recognized for its applications in dye-sensitized solar cells (DSSCs). Beyond its photovoltaic capabilities, this compound exhibits significant biological activity, particularly in the fields of photodynamic therapy (PDT) and as a potential anticancer agent. This article explores its biological mechanisms, efficacy, and relevant case studies.
- Chemical Formula : C26H16N6O8RuS2
- Molecular Weight : 705.6 g/mol
- CAS Number : 141460-19-7
- Appearance : Dark purple solid
- Solubility : Soluble in polar solvents
The biological activity of N3 dye is primarily attributed to its ability to generate reactive oxygen species (ROS) upon light excitation. When exposed to light, the dye undergoes a transition to an excited state, facilitating electron transfer processes that lead to the production of singlet oxygen and other ROS. These reactive species can induce oxidative stress in target cells, contributing to cell death and apoptosis.
1. Photodynamic Therapy (PDT)
N3 dye has been studied for its potential use in PDT, a treatment modality that utilizes photosensitizers to produce cytotoxic species upon light activation. Research indicates that N3 dye can effectively target cancer cells while minimizing damage to surrounding healthy tissues.
2. Anticancer Activity
Studies have demonstrated the anticancer effects of N3 dye against various cancer cell lines, including:
- Breast Cancer (MCF-7) : Exhibited significant cytotoxicity with IC50 values indicating effective dose-response relationships.
- Lung Cancer (A549) : Induced apoptosis through ROS generation and mitochondrial dysfunction.
Case Studies
Study | Cell Line | Light Source | IC50 Value | Mechanism |
---|---|---|---|---|
MCF-7 | LED (660 nm) | 5 µM | ROS-mediated apoptosis | |
A549 | Laser (670 nm) | 3 µM | Mitochondrial disruption | |
HeLa | LED (630 nm) | 4 µM | DNA damage via oxidative stress |
Research Findings
Recent studies have highlighted the following findings regarding the biological activity of N3 dye:
- Enhanced Efficacy with Nanocarriers : The use of nanocarriers has been shown to improve the delivery and efficacy of N3 dye in PDT applications.
- Combination Therapies : Combining N3 dye with traditional chemotherapeutics has yielded synergistic effects, enhancing overall anticancer efficacy.
- Mechanistic Insights : Investigations into the mechanistic pathways have revealed that N3 dye induces apoptosis through both intrinsic and extrinsic pathways, emphasizing its potential as a multi-targeted therapeutic agent.
Q & A
Basic Research Questions
Q. What is the molecular structure and key functional groups of cis-Bis(isothiocyanato)bis(2,2'-bipyridyl-4,4'-dicarboxylato)ruthenium(II) (N3) that contribute to its role as a photosensitizer in DSSCs?
The compound features a ruthenium(II) center coordinated by two bipyridyl-4,4'-dicarboxylato ligands (providing anchoring groups for TiO₂ binding) and two isothiocyanato (NCS⁻) ligands. The carboxylate groups enable chemisorption to TiO₂ surfaces, while the NCS⁻ ligands enhance light absorption and charge-transfer efficiency. The cis-configuration optimizes electron injection into the semiconductor .
Q. How is N3 synthesized and characterized for use in DSSCs?
N3 is synthesized via refluxing RuCl₃ with bipyridyl dicarboxylic acid ligands and thiocyanate under controlled conditions. Characterization involves:
- UV-Vis spectroscopy : Absorption peaks at ~400 nm (metal-to-ligand charge transfer, MLCT) and ~535 nm (ligand-centered transitions) .
- Cyclic voltammetry : Redox potentials (e.g., Ru³⁺/Ru²⁺ at ~0.8 V vs. NHE) confirm stability under operational conditions .
- Luminescence studies : Emission quenching on TiO₂ surfaces validates electron injection efficiency .
Q. What are the primary photophysical properties of N3 that influence its light-harvesting efficiency?
N3 exhibits a broad absorption spectrum (300–800 nm) with a molar extinction coefficient of ~1.4 × 10⁴ M⁻¹cm⁻¹ at 535 nm, enabling strong visible-light absorption. The MLCT transition facilitates electron transfer to TiO₂, while the carboxylate anchoring groups minimize recombination losses .
Advanced Research Questions
Q. How do anchoring groups in N3 affect electron injection efficiency compared to non-anchored Ru complexes?
Anchoring groups (e.g., carboxylate in N3 vs. non-anchored Rubpy) enhance binding to TiO₂, reducing charge recombination. Studies show N3 achieves ~10% efficiency, while Rubpy (without anchoring) exhibits negligible performance due to poor adhesion and rapid electron-hole recombination . Computational DFT studies confirm stronger electronic coupling between N3 and TiO₂ .
Q. What molecular mechanisms govern the regeneration of oxidized N3 by iodide redox mediators?
Molecular dynamics simulations reveal a barrier-free complex formation between oxidized N3 and I⁻/I₃⁻ in acetonitrile. The thiocyanate ligands reversibly bind I₂, facilitating rapid dye regeneration. Solvent structuring near TiO₂ surfaces concentrates I⁻, enhancing regeneration kinetics .
Q. How do structural modifications (e.g., N719 vs. N3) alter electrochemical stability and photovoltaic performance?
N719, the tetrabutylammonium salt of N3, improves solubility and reduces aggregation. Key differences include:
- Higher open-circuit voltage (VOC) : Due to reduced proton-induced charge recombination.
- Efficiency : N719 achieves 11.18% vs. N3’s 10% (AM 1.5G, Table 1) .
- Electrolyte compatibility : N719’s counterions stabilize the dye-electrolyte interface, enhancing long-term stability .
Q. Methodological Recommendations
- Electron Injection Analysis : Use transient absorption spectroscopy to quantify electron injection rates (~fs-ps timescales) .
- Regeneration Studies : Employ in situ Raman spectroscopy to monitor I₃⁻ binding to thiocyanate ligands .
- Comparative Testing : Pair N3 with co-sensitizers (e.g., organic dyes) to broaden spectral response .
Eigenschaften
IUPAC Name |
2-(4-carboxypyridin-2-yl)pyridine-4-carboxylic acid;ruthenium(2+);dithiocyanate | |
---|---|---|
Details | Computed by LexiChem 2.6.6 (PubChem release 2019.06.18) | |
Source | PubChem | |
URL | https://pubchem.ncbi.nlm.nih.gov | |
Description | Data deposited in or computed by PubChem | |
InChI |
InChI=1S/2C12H8N2O4.2CHNS.Ru/c2*15-11(16)7-1-3-13-9(5-7)10-6-8(12(17)18)2-4-14-10;2*2-1-3;/h2*1-6H,(H,15,16)(H,17,18);2*3H;/q;;;;+2/p-2 | |
Details | Computed by InChI 1.0.5 (PubChem release 2019.06.18) | |
Source | PubChem | |
URL | https://pubchem.ncbi.nlm.nih.gov | |
Description | Data deposited in or computed by PubChem | |
InChI Key |
LTNAYKNIZNSHQA-UHFFFAOYSA-L | |
Details | Computed by InChI 1.0.5 (PubChem release 2019.06.18) | |
Source | PubChem | |
URL | https://pubchem.ncbi.nlm.nih.gov | |
Description | Data deposited in or computed by PubChem | |
Canonical SMILES |
C1=CN=C(C=C1C(=O)O)C2=NC=CC(=C2)C(=O)O.C1=CN=C(C=C1C(=O)O)C2=NC=CC(=C2)C(=O)O.C(#N)[S-].C(#N)[S-].[Ru+2] | |
Details | Computed by OEChem 2.1.5 (PubChem release 2019.06.18) | |
Source | PubChem | |
URL | https://pubchem.ncbi.nlm.nih.gov | |
Description | Data deposited in or computed by PubChem | |
Molecular Formula |
C26H16N6O8RuS2 | |
Details | Computed by PubChem 2.1 (PubChem release 2019.06.18) | |
Source | PubChem | |
URL | https://pubchem.ncbi.nlm.nih.gov | |
Description | Data deposited in or computed by PubChem | |
Molecular Weight |
705.6 g/mol | |
Details | Computed by PubChem 2.1 (PubChem release 2021.05.07) | |
Source | PubChem | |
URL | https://pubchem.ncbi.nlm.nih.gov | |
Description | Data deposited in or computed by PubChem | |
CAS No. |
141460-19-7 | |
Record name | Bis(isothiocyanato)bis(2,2'-bipyridyl-4,4'-dicarboxylato)ruthenium(II) | |
Source | European Chemicals Agency (ECHA) | |
URL | https://echa.europa.eu/information-on-chemicals | |
Description | The European Chemicals Agency (ECHA) is an agency of the European Union which is the driving force among regulatory authorities in implementing the EU's groundbreaking chemicals legislation for the benefit of human health and the environment as well as for innovation and competitiveness. | |
Explanation | Use of the information, documents and data from the ECHA website is subject to the terms and conditions of this Legal Notice, and subject to other binding limitations provided for under applicable law, the information, documents and data made available on the ECHA website may be reproduced, distributed and/or used, totally or in part, for non-commercial purposes provided that ECHA is acknowledged as the source: "Source: European Chemicals Agency, http://echa.europa.eu/". Such acknowledgement must be included in each copy of the material. ECHA permits and encourages organisations and individuals to create links to the ECHA website under the following cumulative conditions: Links can only be made to webpages that provide a link to the Legal Notice page. | |
Retrosynthesis Analysis
AI-Powered Synthesis Planning: Our tool employs the Template_relevance Pistachio, Template_relevance Bkms_metabolic, Template_relevance Pistachio_ringbreaker, Template_relevance Reaxys, Template_relevance Reaxys_biocatalysis model, leveraging a vast database of chemical reactions to predict feasible synthetic routes.
One-Step Synthesis Focus: Specifically designed for one-step synthesis, it provides concise and direct routes for your target compounds, streamlining the synthesis process.
Accurate Predictions: Utilizing the extensive PISTACHIO, BKMS_METABOLIC, PISTACHIO_RINGBREAKER, REAXYS, REAXYS_BIOCATALYSIS database, our tool offers high-accuracy predictions, reflecting the latest in chemical research and data.
Strategy Settings
Precursor scoring | Relevance Heuristic |
---|---|
Min. plausibility | 0.01 |
Model | Template_relevance |
Template Set | Pistachio/Bkms_metabolic/Pistachio_ringbreaker/Reaxys/Reaxys_biocatalysis |
Top-N result to add to graph | 6 |
Feasible Synthetic Routes
Haftungsausschluss und Informationen zu In-Vitro-Forschungsprodukten
Bitte beachten Sie, dass alle Artikel und Produktinformationen, die auf BenchChem präsentiert werden, ausschließlich zu Informationszwecken bestimmt sind. Die auf BenchChem zum Kauf angebotenen Produkte sind speziell für In-vitro-Studien konzipiert, die außerhalb lebender Organismen durchgeführt werden. In-vitro-Studien, abgeleitet von dem lateinischen Begriff "in Glas", beinhalten Experimente, die in kontrollierten Laborumgebungen unter Verwendung von Zellen oder Geweben durchgeführt werden. Es ist wichtig zu beachten, dass diese Produkte nicht als Arzneimittel oder Medikamente eingestuft sind und keine Zulassung der FDA für die Vorbeugung, Behandlung oder Heilung von medizinischen Zuständen, Beschwerden oder Krankheiten erhalten haben. Wir müssen betonen, dass jede Form der körperlichen Einführung dieser Produkte in Menschen oder Tiere gesetzlich strikt untersagt ist. Es ist unerlässlich, sich an diese Richtlinien zu halten, um die Einhaltung rechtlicher und ethischer Standards in Forschung und Experiment zu gewährleisten.