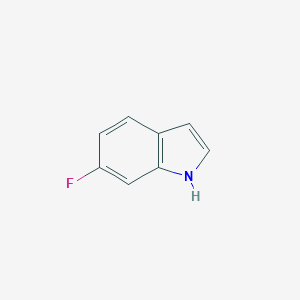
6-Fluoroindole
Übersicht
Beschreibung
6-Fluoroindole is a halogen-substituted indole, characterized by the presence of a fluorine atom at the sixth position of the indole ring.
Wirkmechanismus
Target of Action
6-Fluoroindole, also known as 6-fluoro-1H-indole, primarily targets tubulin in colorectal cancer (CRC) cells . Tubulin is a globular protein and is the main component of microtubules in cells. These microtubules are crucial for various cellular functions, including maintenance of cell shape, intracellular transport, and cell division .
Mode of Action
This compound interacts with its target, tubulin, and induces G2/M phase arrest by regulating cyclin B1 expression . Cyclin B1 is a regulatory protein involved in mitosis, and its expression is necessary for the cell to progress from the G2 phase to the M phase of the cell cycle . By regulating cyclin B1 expression, this compound can halt the cell cycle, preventing the cells from dividing and proliferating .
Biochemical Pathways
The primary biochemical pathway affected by this compound is the cell cycle, specifically the transition from the G2 phase to the M phase . By inducing G2/M phase arrest, this compound disrupts the normal progression of the cell cycle, leading to a decrease in cell proliferation . This disruption can lead to cell death, particularly in cancer cells that rely on rapid cell division for growth .
Result of Action
The result of this compound’s action is a reduction in the proliferation of CRC cells . By inducing G2/M phase arrest and producing excess reactive oxygen species (ROS), this compound can lead to cell death . This makes it a potential candidate for the development of anticancer drugs .
Biochemische Analyse
Biochemical Properties
6-Fluoroindole interacts with various enzymes, proteins, and other biomolecules. It can be easily functionalized with Bpin groups (pinacol boronate) at 2-, 3-, 4-, 5- and 7-positions selectively with iridium-catalysed borylation and bismuth-catalysed protodeboronation . This modification allows late-stage functionalization on different positions of the molecule, expanding the molecular library for drug discovery .
Cellular Effects
This compound has been shown to have significant effects on various types of cells and cellular processes. For instance, it has been found to inhibit biofilm formation, a key factor in the development of many bacterial infections . In Escherichia coli, this compound has been shown to interfere with the quorum sensing system of the pathogens .
Molecular Mechanism
The molecular mechanism of this compound involves its interaction with the quorum sensing system of pathogens, thereby inhibiting biofilm formation . In adaptive laboratory evolution (ALE) experiments, this compound was converted into the corresponding amino acids (6-fluorotryptophan) within the cells and globally incorporated into the proteome at tryptophan sites .
Temporal Effects in Laboratory Settings
Over time, in laboratory settings, this compound has been shown to have a significant impact on the growth of microbial cells. In ALE experiments, Escherichia coli evolved to utilize this compound for growth .
Metabolic Pathways
In metabolic pathways, this compound is converted into 6-fluorotryptophan within the cells . This conversion is a part of the adaptive response of the cells to the presence of this compound.
Vorbereitungsmethoden
Synthetic Routes and Reaction Conditions: 6-Fluoroindole can be synthesized through several methods. One common approach involves the nitration of indoline, followed by reduction and cyclization to yield the desired product . Another method includes the electrophilic fluorination of indole derivatives using reagents such as trifluoromethyl hypofluorite or cesium fluoroxysulfate .
Industrial Production Methods: While laboratory-scale synthesis of this compound is well-documented, large-scale industrial production methods are less common. the scalability of the nitration and fluorination processes suggests potential for industrial application, provided that cost-effective and environmentally friendly conditions are optimized .
Analyse Chemischer Reaktionen
Types of Reactions: 6-Fluoroindole undergoes various chemical reactions, including:
Electrophilic Substitution: The fluorine atom’s electron-withdrawing nature makes the indole ring more reactive towards electrophiles.
Nucleophilic Substitution: The fluorine atom can be replaced by other nucleophiles under appropriate conditions.
Oxidation and Reduction: this compound can be oxidized or reduced to form different derivatives
Common Reagents and Conditions:
Electrophilic Substitution: Reagents such as trifluoromethyl hypofluorite and cesium fluoroxysulfate are commonly used.
Nucleophilic Substitution: Nucleophiles like amines and thiols can be employed.
Oxidation and Reduction: Oxidizing agents like potassium permanganate and reducing agents like lithium aluminum hydride are used.
Major Products: The reactions of this compound typically yield a variety of substituted indoles, which can be further functionalized for specific applications .
Wissenschaftliche Forschungsanwendungen
6-Fluoroindole has a wide range of applications in scientific research:
Medicinal Chemistry: It is used in the synthesis of tryptophan dioxygenase inhibitors, potential anticancer immunomodulators, and selective serotonin reuptake inhibitors
Material Science: It serves as a building block for organic light-emitting diodes (OLEDs), semiconductors, and dye-sensitized solar cells (DSSCs).
Antimicrobial Agents: The compound exhibits antibacterial and antifungal properties, making it useful in developing new antimicrobial agents.
Vergleich Mit ähnlichen Verbindungen
6-Fluoroindole can be compared with other fluorinated indoles and halogen-substituted indoles:
Eigenschaften
IUPAC Name |
6-fluoro-1H-indole | |
---|---|---|
Source | PubChem | |
URL | https://pubchem.ncbi.nlm.nih.gov | |
Description | Data deposited in or computed by PubChem | |
InChI |
InChI=1S/C8H6FN/c9-7-2-1-6-3-4-10-8(6)5-7/h1-5,10H | |
Source | PubChem | |
URL | https://pubchem.ncbi.nlm.nih.gov | |
Description | Data deposited in or computed by PubChem | |
InChI Key |
YYFFEPUCAKVRJX-UHFFFAOYSA-N | |
Source | PubChem | |
URL | https://pubchem.ncbi.nlm.nih.gov | |
Description | Data deposited in or computed by PubChem | |
Canonical SMILES |
C1=CC(=CC2=C1C=CN2)F | |
Source | PubChem | |
URL | https://pubchem.ncbi.nlm.nih.gov | |
Description | Data deposited in or computed by PubChem | |
Molecular Formula |
C8H6FN | |
Source | PubChem | |
URL | https://pubchem.ncbi.nlm.nih.gov | |
Description | Data deposited in or computed by PubChem | |
DSSTOX Substance ID |
DTXSID10325857 | |
Record name | 6-Fluoroindole | |
Source | EPA DSSTox | |
URL | https://comptox.epa.gov/dashboard/DTXSID10325857 | |
Description | DSSTox provides a high quality public chemistry resource for supporting improved predictive toxicology. | |
Molecular Weight |
135.14 g/mol | |
Source | PubChem | |
URL | https://pubchem.ncbi.nlm.nih.gov | |
Description | Data deposited in or computed by PubChem | |
CAS No. |
399-51-9 | |
Record name | 399-51-9 | |
Source | DTP/NCI | |
URL | https://dtp.cancer.gov/dtpstandard/servlet/dwindex?searchtype=NSC&outputformat=html&searchlist=520436 | |
Description | The NCI Development Therapeutics Program (DTP) provides services and resources to the academic and private-sector research communities worldwide to facilitate the discovery and development of new cancer therapeutic agents. | |
Explanation | Unless otherwise indicated, all text within NCI products is free of copyright and may be reused without our permission. Credit the National Cancer Institute as the source. | |
Record name | 6-Fluoroindole | |
Source | EPA DSSTox | |
URL | https://comptox.epa.gov/dashboard/DTXSID10325857 | |
Description | DSSTox provides a high quality public chemistry resource for supporting improved predictive toxicology. | |
Record name | 6-Fluoroindole | |
Source | European Chemicals Agency (ECHA) | |
URL | https://echa.europa.eu/information-on-chemicals | |
Description | The European Chemicals Agency (ECHA) is an agency of the European Union which is the driving force among regulatory authorities in implementing the EU's groundbreaking chemicals legislation for the benefit of human health and the environment as well as for innovation and competitiveness. | |
Explanation | Use of the information, documents and data from the ECHA website is subject to the terms and conditions of this Legal Notice, and subject to other binding limitations provided for under applicable law, the information, documents and data made available on the ECHA website may be reproduced, distributed and/or used, totally or in part, for non-commercial purposes provided that ECHA is acknowledged as the source: "Source: European Chemicals Agency, http://echa.europa.eu/". Such acknowledgement must be included in each copy of the material. ECHA permits and encourages organisations and individuals to create links to the ECHA website under the following cumulative conditions: Links can only be made to webpages that provide a link to the Legal Notice page. | |
Synthesis routes and methods I
Procedure details
Synthesis routes and methods II
Procedure details
Retrosynthesis Analysis
AI-Powered Synthesis Planning: Our tool employs the Template_relevance Pistachio, Template_relevance Bkms_metabolic, Template_relevance Pistachio_ringbreaker, Template_relevance Reaxys, Template_relevance Reaxys_biocatalysis model, leveraging a vast database of chemical reactions to predict feasible synthetic routes.
One-Step Synthesis Focus: Specifically designed for one-step synthesis, it provides concise and direct routes for your target compounds, streamlining the synthesis process.
Accurate Predictions: Utilizing the extensive PISTACHIO, BKMS_METABOLIC, PISTACHIO_RINGBREAKER, REAXYS, REAXYS_BIOCATALYSIS database, our tool offers high-accuracy predictions, reflecting the latest in chemical research and data.
Strategy Settings
Precursor scoring | Relevance Heuristic |
---|---|
Min. plausibility | 0.01 |
Model | Template_relevance |
Template Set | Pistachio/Bkms_metabolic/Pistachio_ringbreaker/Reaxys/Reaxys_biocatalysis |
Top-N result to add to graph | 6 |
Feasible Synthetic Routes
Q1: What is the molecular formula and weight of 6-fluoroindole?
A1: this compound has a molecular formula of C8H6FN and a molecular weight of 135.14 g/mol.
Q2: Is there any spectroscopic data available for this compound?
A2: Yes, researchers have conducted various spectroscopic studies on this compound.
- Vibronic Analysis: Rotationally resolved electronic Stark spectroscopy coupled with high-level ab initio calculations was used to characterize the lowest two electronically excited singlet states (La and Lb) of this compound. This research revealed that changing the fluorine atom's position from the 5- to the 6-position increases La character in the S1 state, impacting the transition dipole moment and challenging the traditional La/Lb nomenclature due to heavily mixed excited states. []
- Electronic Absorption Spectra: Studies have investigated the electronic absorption spectra of this compound, providing insights into its electronic structure and transitions. []
Q3: Does this compound possess any catalytic properties?
A3: The provided research doesn't focus on the catalytic properties of this compound. It primarily explores its role as a substrate or intermediate in biological and chemical synthesis.
Q4: Have computational methods been employed to study this compound?
A4: Yes, computational chemistry has played a significant role in understanding this compound.
- Ionization Potential Calculations: Ab initio calculations accurately predicted the ionization potentials (IPs) of this compound and related indole derivatives. These calculations revealed that 6-fluoro substitution increases the IP of indole by 0.09 eV. [, ]
- Vibronic Analysis: High-level ab initio calculations, combined with spectroscopic data, helped analyze the vibronic structure and excited states of this compound. []
Q5: How does the fluorine atom at the 6-position influence the activity of indole?
A5: The position of the fluorine atom on the indole ring significantly impacts its biological activity.
- Tryptophan Synthase Interaction: this compound acts as a substrate for tryptophan synthase, leading to the production of 6-fluorotryptophan. [, , ]
- Quorum Sensing Inhibition: Research demonstrates that this compound interferes with quorum sensing in Serratia marcescens, impacting biofilm formation and virulence factor production. []
Q6: Are there any specific formulation strategies mentioned for this compound?
A6: The provided research primarily focuses on the synthesis and biological evaluation of this compound. There isn't detailed information regarding specific formulation strategies.
Q7: Is there any information regarding the SHE regulations concerning this compound?
A7: The provided articles primarily focus on the scientific aspects of this compound and do not delve into specific SHE regulations. As with any chemical, it's crucial to handle this compound with appropriate safety measures and consult relevant safety data sheets.
Q8: What is known about the absorption, distribution, metabolism, and excretion of this compound?
A9: Although the research doesn't provide detailed PK/PD data for this compound itself, it sheds light on its metabolic fate. Studies show that Escherichia coli can metabolize this compound into 6-fluorotryptophan, which is then incorporated into proteins. [, ] This suggests potential metabolic pathways for this compound.
Q9: Has this compound shown efficacy in any in vitro or in vivo models?
A9: Yes, studies highlight the biological activity of this compound:
- Antibiofilm and Antivirulence Activity: this compound demonstrates significant antibiofilm and antivirulence properties against Serratia marcescens in in vitro assays. []
- Toluene Dioxygenase Induction: Research shows that this compound acts as a substrate for toluene dioxygenase in Pseudomonas putida F1, inducing its own metabolism and leading to the formation of fluorescent 6-fluoroindoxyl. []
- Adaptive Laboratory Evolution: Through adaptive laboratory evolution, Escherichia coli strains have been developed that can utilize this compound for growth, showcasing its potential as a substrate for bacterial metabolism. [, ]
Haftungsausschluss und Informationen zu In-Vitro-Forschungsprodukten
Bitte beachten Sie, dass alle Artikel und Produktinformationen, die auf BenchChem präsentiert werden, ausschließlich zu Informationszwecken bestimmt sind. Die auf BenchChem zum Kauf angebotenen Produkte sind speziell für In-vitro-Studien konzipiert, die außerhalb lebender Organismen durchgeführt werden. In-vitro-Studien, abgeleitet von dem lateinischen Begriff "in Glas", beinhalten Experimente, die in kontrollierten Laborumgebungen unter Verwendung von Zellen oder Geweben durchgeführt werden. Es ist wichtig zu beachten, dass diese Produkte nicht als Arzneimittel oder Medikamente eingestuft sind und keine Zulassung der FDA für die Vorbeugung, Behandlung oder Heilung von medizinischen Zuständen, Beschwerden oder Krankheiten erhalten haben. Wir müssen betonen, dass jede Form der körperlichen Einführung dieser Produkte in Menschen oder Tiere gesetzlich strikt untersagt ist. Es ist unerlässlich, sich an diese Richtlinien zu halten, um die Einhaltung rechtlicher und ethischer Standards in Forschung und Experiment zu gewährleisten.