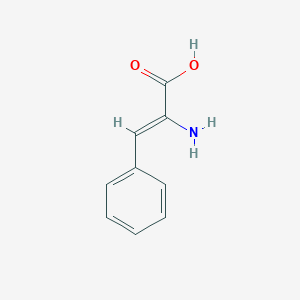
Phenyldehydroalanine
Übersicht
Beschreibung
Phenyldehydroalanine (systematic name: Acetyl-α,β-dehydro-phenylalanine) is a non-proteinogenic amino acid derivative characterized by an α,β-unsaturated carbonyl group (dehydroalanine backbone) conjugated to a phenyl moiety. Its molecular formula is C₁₁H₁₁NO₃ (CAS# 5469-45-4), and it is primarily utilized in research settings for studying peptide cross-linking, enzyme mechanisms, and reactive intermediates . The α,β-unsaturation confers electrophilic reactivity, making it prone to nucleophilic additions and participation in Michael reactions, which is critical in protein modification studies .
Vorbereitungsmethoden
Synthetic Routes and Reaction Conditions
Phenyldehydroalanine can be synthesized through several methods. One common approach involves the preparation of phenylselenocysteine, which is then converted to this compound through a chemoselective, mild oxidation process using hydrogen peroxide or sodium periodate . This method allows for the incorporation of this compound into peptides by standard solid-phase peptide synthesis techniques.
Another method involves the use of alpha-bromo-phenylalanine, which undergoes dehydrohalogenation to form this compound. This reaction typically requires a strong base such as sodium hydroxide or potassium tert-butoxide .
Industrial Production Methods
Industrial production of this compound is less common due to its specialized applications. the methods mentioned above can be scaled up for larger production if needed. The choice of method depends on the desired purity and yield of the final product.
Analyse Chemischer Reaktionen
Acylation Reactions
PDA serves as a probe for enzyme acylation mechanisms:
-
Papain-Catalyzed Acylation :
PDA-containing peptides (e.g., Ac-Phe-Phe-PDA-OEt) undergo acylation at papain’s active site, inducing a 49 nm red shift (277 → 326 nm) due to chromophore environmental changes .
Alkylation and Crosslinking
PDA derivatives participate in nucleophilic additions:
-
Lysinoalanine Formation :
Reacting PDA esters (e.g., phenylacetamido acrylic acid methyl ester) with proteins at pH 9–10 converts lysine residues to lysinoalanine .
Michael Addition Reactions
The α,β-unsaturated system undergoes conjugate additions:
-
Thiol Additions :
PDA reacts with cysteine thiols in peptides, forming stable adducts. This reaction is critical for probing redox-sensitive enzymatic sites .
Enzymatic Interactions
PDA’s reactivity is exploited to study enzyme mechanisms:
-
Papain Binding :
Noncovalent PDA-peptide binding to papain alters absorption spectra of auxiliary chromophores (e.g., DAP at 480 nm) . -
Protease Specificity :
PDA-containing inhibitors (e.g., Ala-Ala-Phe-SDA) exhibit K<sub>i</sub> = 2.9 × 10<sup>−5</sup> M, highlighting steric and electronic effects on enzyme affinity .
Table 1: Key Reaction Parameters of Phenyldehydroalanine
Mechanistic Insights
-
Spectroscopic Utility : PDA’s chromophoric shifts (Δλ = 49–59 nm) enable real-time monitoring of acylation and binding events .
-
pH Dependence : Reaction rates correlate with protonation states of catalytic residues (e.g., papain’s Cys-25) .
-
Steric Effects : Bulky PDA side chains hinder enzyme active-site access, altering kinetic parameters .
This compound’s versatility in probing enzymatic mechanisms and protein modifications underscores its value in biochemical research. Future applications may leverage its photophysical properties for advanced imaging or targeted drug design.
Wissenschaftliche Forschungsanwendungen
Chemical Synthesis
Phenyldehydroalanine is utilized as a precursor in the synthesis of peptides and other complex organic molecules. Its unique structure allows for the incorporation into peptide chains, which can lead to novel conformations and biological activities.
Peptide Synthesis
Research indicates that peptides containing (Z)-β-Phenyldehydroalanine exhibit interesting conformational properties. For example, a linear hexapeptide with two ΔZPhe residues was found to adopt a looping backbone structure stabilized by intramolecular hydrogen bonds, showcasing the compound's impact on peptide conformation .
Biological Applications
In biological research, PDA-containing peptides are studied for their potential therapeutic effects. They have been investigated for their biological activities, including enzyme inhibition and modulation of protein interactions.
Enzyme Mechanism Studies
PDA serves as a valuable tool for studying enzyme mechanisms, particularly cysteine proteases like papain. The compound's ability to undergo acylation results in significant changes in UV absorbance spectra, allowing researchers to monitor the kinetics of enzyme activity directly . This property has made it essential in elucidating catalytic mechanisms.
Antiproliferative Activity
Recent studies have explored the antiproliferative effects of PDA-containing peptides against various cancer cell lines. For instance, dipeptides synthesized from S-substituted dehydrocysteine derivatives exhibited promising antiproliferative activity, suggesting potential applications in cancer therapy .
Pharmaceutical Applications
Pharmaceutical research is increasingly focusing on bioactive peptides derived from PDA for their therapeutic potential. These peptides have been identified as having anti-inflammatory, anticancer, and antidiabetic properties.
Bioactive Peptides
Bioactive peptides that include modifications like PDA are being developed for use as pharmaceutical agents. Their ability to modulate biological processes makes them candidates for drug development aimed at treating metabolic disorders and cancers .
Case Studies
The following table summarizes notable case studies involving this compound and its derivatives:
Wirkmechanismus
Phenyldehydroalanine exerts its effects through its electrophilic double bond, which makes it a valuable precursor for peptide conjugates via intermolecular Michael-type addition. This electrophilicity allows it to react with nucleophiles, forming stable conjugates. The molecular targets and pathways involved depend on the specific application and the nature of the nucleophile used .
Vergleich Mit ähnlichen Verbindungen
Comparative Analysis with Structurally Related Compounds
3-(2,5-Cyclohexadienyl)-L-alanine (1,4-Dihydro-L-phenylalanine)
- Structure : Features a cyclohexadienyl ring instead of a benzene ring, introducing partial saturation and altered conjugation.
- Synthesis : Synthesized via enzymatic reactions using phenylalanine ammonia-lyase (PAL), where the aromatic ring undergoes partial reduction .
- Reactivity : The dienyl structure allows for unique interactions in enzyme active sites, particularly in PAL-mediated deamination studies. Unlike Phenyldehydroalanine, it lacks the α,β-unsaturated carbonyl, reducing electrophilicity .
Dihydroxyphenylalanine (DOPA)
- Structure: Contains two hydroxyl groups on the phenyl ring (C₉H₁₁NO₄; CAS# 63-84-3), enhancing polarity and redox activity .
- Biological Role : A precursor to dopamine and melanin, widely used in Parkinson’s disease treatment. Unlike this compound, DOPA’s catechol group facilitates metal chelation and oxidative polymerization .
- Synthesis : Produced via tyrosine hydroxylation in vivo or via chemical synthesis using hydroxylation catalysts .
Thienylalanine
- Structure : Replaces the benzene ring with a thiophene (sulfur-containing heterocycle), altering electronic properties and metabolic stability .
- Applications: Used in studies exploring non-canonical amino acid incorporation into proteins. The thiophene’s lower electron density reduces aromatic interactions compared to this compound .
N-Phthaloyl-L-phenylalanine
- Structure: Phthaloyl-protected phenylalanine (C₁₈H₁₅NO₄), with a bulky phthalimide group enhancing steric hindrance .
- Use in Synthesis : Employed in peptide synthesis to block amine groups. Contrasts with this compound’s reactive dehydroalanine moiety, which is used for cross-linking rather than protection .
3-(2-Fluoro-18F-4,5-dihydroxy-phenyl)-L-alanine
- Structure : Radiolabeled fluorinated derivative of DOPA (CAS# 92812-82-3), used in positron emission tomography (PET) imaging .
- Function: Combines fluorine’s isotopic properties with DOPA’s biochemical pathway, enabling neuroimaging. Unlike this compound, this compound emphasizes diagnostic over reactive applications .
Structural and Functional Comparison Table
Key Research Findings
- Reactivity : this compound’s α,β-unsaturated system enables selective modifications in peptides, unlike DOPA’s redox-driven polymerization .
- Enzyme Interactions : 1,4-Dihydro-L-phenylalanine’s dienyl structure mimics transition states in PAL-catalyzed reactions, whereas this compound acts as an electrophilic trap .
- Metabolic Pathways : Thienylalanine avoids typical phenylalanine metabolism, highlighting the impact of aromatic substitution on biochemical fate .
Biologische Aktivität
Phenyldehydroalanine (PDA) is a modified amino acid that has garnered interest due to its diverse biological activities, particularly in the fields of immunology and cancer research. This article provides a comprehensive overview of the biological activity of PDA, including its mechanisms of action, potential therapeutic applications, and relevant case studies.
Structural Characteristics
This compound is a structural analog of phenylalanine, characterized by the presence of a double bond between the alpha and beta carbon atoms. This modification significantly alters its biochemical properties and interactions with biological macromolecules.
PDA exhibits multiple mechanisms of action that contribute to its biological effects:
- NOD2 Agonism : PDA has been identified as a potent agonist for NOD2, a pattern recognition receptor involved in immune response. The effective concentration (EC50) for NOD2 activation is reported to be 46 nM, highlighting its potential as an immunomodulatory agent .
- Cytokine Release : In human peripheral blood mononuclear cells, PDA enhances the release of pro-inflammatory cytokines when combined with lipopolysaccharides (LPS). This suggests a role in amplifying immune responses during infections .
- Anticancer Activity : PDA derivatives have shown promising anticancer properties. In vitro studies indicate that certain PDA-based compounds exhibit cytotoxic effects on various cancer cell lines, with IC50 values ranging from 2.1 to 7.9 μM. These effects are attributed to mechanisms such as apoptosis induction and cell cycle arrest .
Biological Activity Summary
The biological activities associated with PDA can be summarized as follows:
Case Studies
Several studies have explored the biological activity of PDA and its derivatives:
- Immunological Response : In a study examining the effects of PDA on immune cells, it was found that PDA significantly increased the production of TNF-α and IL-6 in response to bacterial components, indicating its role as an immunomodulator .
- Cancer Cell Line Studies : A series of experiments conducted on various cancer cell lines revealed that PDA derivatives not only inhibited cell proliferation but also induced apoptosis through mitochondrial pathways, suggesting potential for therapeutic development in oncology .
- Antimicrobial Research : Research into the antimicrobial properties of PDA indicated effective inhibition against several bacteria, with minimum inhibitory concentrations (MIC) showing promising results against both gram-positive and gram-negative strains .
Q & A
Basic Research Questions
Q. What are the established methods for synthesizing Phenyldehydroalanine, and how can researchers optimize reaction conditions?
Answer: this compound synthesis typically involves dehydration or elimination reactions of phenylalanine derivatives. To optimize conditions, systematically vary parameters (e.g., temperature, pH, catalysts) and monitor yields via HPLC or mass spectrometry. Include controls to isolate variables and validate reproducibility across trials. Detailed protocols should specify reagent purity, solvent systems, and purification steps to ensure consistency .
Q. Which spectroscopic and chromatographic techniques are most reliable for characterizing Phenyldihydroalanine’s structure and purity?
Answer: Nuclear Magnetic Resonance (NMR) spectroscopy (1H/13C) is critical for structural confirmation, while High-Resolution Mass Spectrometry (HRMS) validates molecular weight. Pair these with reverse-phase HPLC to assess purity. For quantitative analysis, use calibration curves with certified reference standards. Report solvent systems, column types, and detection wavelengths to enhance reproducibility .
Q. How should researchers design stability studies for this compound under varying storage conditions?
Answer: Conduct accelerated stability testing by exposing the compound to controlled stressors (light, humidity, temperature). Use LC-MS to track degradation products and kinetic modeling (e.g., Arrhenius plots) to predict shelf life. Include triplicate samples and statistical analysis (e.g., ANOVA) to assess significance of observed changes. Document deviations from ICH guidelines if applicable .
Advanced Research Questions
Q. How can computational methods resolve contradictions in reported electronic properties of this compound?
Answer: Perform density functional theory (DFT) calculations to model the compound’s electron distribution and compare results with experimental data (e.g., X-ray crystallography, UV-Vis spectra). Address discrepancies by refining computational parameters (basis sets, solvation models) or re-evaluating experimental conditions (e.g., crystal packing effects). Cross-validate findings with independent techniques like cyclic voltammetry .
Q. What strategies are effective for analyzing the mechanistic role of this compound in peptide crosslinking or oxidation pathways?
Answer: Use isotopic labeling (e.g., 18O or 2H) to track reaction intermediates via tandem MS. Combine stopped-flow kinetics with time-resolved spectroscopy to identify transient species. For biological systems, employ site-directed mutagenesis to isolate the compound’s interactions with specific amino acid residues. Contrast results with control experiments using non-reactive analogs .
Q. How should researchers address conflicting data on this compound’s reactivity in different solvent systems?
Answer: Apply multivariate analysis (e.g., PCA) to identify solvent properties (polarity, dielectric constant) correlating with reactivity trends. Validate hypotheses using solvent-free conditions (e.g., ball milling) or ionic liquids. Publish raw datasets and statistical codes to enable peer verification. Discuss limitations of current models (e.g., neglect of steric effects) and propose follow-up studies .
Q. What experimental frameworks are suitable for investigating this compound’s role in post-translational modifications in vivo?
Answer: Develop a knockout model (e.g., CRISPR/Cas9) to disable endogenous pathways producing the compound. Use immunofluorescence or click chemistry probes to visualize its localization. Pair this with metabolomic profiling (LC-MS/MS) to identify associated biomarkers. Ensure ethical compliance by submitting protocols for institutional review .
Q. Methodological Best Practices
- Data Presentation : Organize results using tables comparing synthetic yields, spectral peaks, or kinetic constants. Highlight anomalies in supplementary materials to maintain clarity .
- Contradiction Analysis : Apply the FINER criteria (Feasible, Interesting, Novel, Ethical, Relevant) to prioritize unresolved questions .
- Reproducibility : Adhere to FAIR principles (Findable, Accessible, Interoperable, Reusable) by depositing raw data in repositories like Zenodo or ChemRxiv .
Eigenschaften
IUPAC Name |
(Z)-2-amino-3-phenylprop-2-enoic acid | |
---|---|---|
Source | PubChem | |
URL | https://pubchem.ncbi.nlm.nih.gov | |
Description | Data deposited in or computed by PubChem | |
InChI |
InChI=1S/C9H9NO2/c10-8(9(11)12)6-7-4-2-1-3-5-7/h1-6H,10H2,(H,11,12)/b8-6- | |
Source | PubChem | |
URL | https://pubchem.ncbi.nlm.nih.gov | |
Description | Data deposited in or computed by PubChem | |
InChI Key |
YWIQQKOKNPPGDO-VURMDHGXSA-N | |
Source | PubChem | |
URL | https://pubchem.ncbi.nlm.nih.gov | |
Description | Data deposited in or computed by PubChem | |
Canonical SMILES |
C1=CC=C(C=C1)C=C(C(=O)O)N | |
Source | PubChem | |
URL | https://pubchem.ncbi.nlm.nih.gov | |
Description | Data deposited in or computed by PubChem | |
Isomeric SMILES |
C1=CC=C(C=C1)/C=C(/C(=O)O)\N | |
Source | PubChem | |
URL | https://pubchem.ncbi.nlm.nih.gov | |
Description | Data deposited in or computed by PubChem | |
Molecular Formula |
C9H9NO2 | |
Source | PubChem | |
URL | https://pubchem.ncbi.nlm.nih.gov | |
Description | Data deposited in or computed by PubChem | |
Molecular Weight |
163.17 g/mol | |
Source | PubChem | |
URL | https://pubchem.ncbi.nlm.nih.gov | |
Description | Data deposited in or computed by PubChem | |
CAS No. |
7060-39-1 | |
Record name | Phenyldehydroalanine | |
Source | ChemIDplus | |
URL | https://pubchem.ncbi.nlm.nih.gov/substance/?source=chemidplus&sourceid=0007060391 | |
Description | ChemIDplus is a free, web search system that provides access to the structure and nomenclature authority files used for the identification of chemical substances cited in National Library of Medicine (NLM) databases, including the TOXNET system. | |
Record name | Phenyldehydroalanine | |
Source | DrugBank | |
URL | https://www.drugbank.ca/drugs/DB04584 | |
Description | The DrugBank database is a unique bioinformatics and cheminformatics resource that combines detailed drug (i.e. chemical, pharmacological and pharmaceutical) data with comprehensive drug target (i.e. sequence, structure, and pathway) information. | |
Explanation | Creative Common's Attribution-NonCommercial 4.0 International License (http://creativecommons.org/licenses/by-nc/4.0/legalcode) | |
Retrosynthesis Analysis
AI-Powered Synthesis Planning: Our tool employs the Template_relevance Pistachio, Template_relevance Bkms_metabolic, Template_relevance Pistachio_ringbreaker, Template_relevance Reaxys, Template_relevance Reaxys_biocatalysis model, leveraging a vast database of chemical reactions to predict feasible synthetic routes.
One-Step Synthesis Focus: Specifically designed for one-step synthesis, it provides concise and direct routes for your target compounds, streamlining the synthesis process.
Accurate Predictions: Utilizing the extensive PISTACHIO, BKMS_METABOLIC, PISTACHIO_RINGBREAKER, REAXYS, REAXYS_BIOCATALYSIS database, our tool offers high-accuracy predictions, reflecting the latest in chemical research and data.
Strategy Settings
Precursor scoring | Relevance Heuristic |
---|---|
Min. plausibility | 0.01 |
Model | Template_relevance |
Template Set | Pistachio/Bkms_metabolic/Pistachio_ringbreaker/Reaxys/Reaxys_biocatalysis |
Top-N result to add to graph | 6 |
Feasible Synthetic Routes
Haftungsausschluss und Informationen zu In-Vitro-Forschungsprodukten
Bitte beachten Sie, dass alle Artikel und Produktinformationen, die auf BenchChem präsentiert werden, ausschließlich zu Informationszwecken bestimmt sind. Die auf BenchChem zum Kauf angebotenen Produkte sind speziell für In-vitro-Studien konzipiert, die außerhalb lebender Organismen durchgeführt werden. In-vitro-Studien, abgeleitet von dem lateinischen Begriff "in Glas", beinhalten Experimente, die in kontrollierten Laborumgebungen unter Verwendung von Zellen oder Geweben durchgeführt werden. Es ist wichtig zu beachten, dass diese Produkte nicht als Arzneimittel oder Medikamente eingestuft sind und keine Zulassung der FDA für die Vorbeugung, Behandlung oder Heilung von medizinischen Zuständen, Beschwerden oder Krankheiten erhalten haben. Wir müssen betonen, dass jede Form der körperlichen Einführung dieser Produkte in Menschen oder Tiere gesetzlich strikt untersagt ist. Es ist unerlässlich, sich an diese Richtlinien zu halten, um die Einhaltung rechtlicher und ethischer Standards in Forschung und Experiment zu gewährleisten.