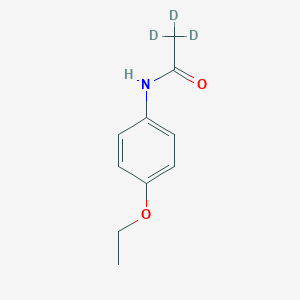
Phenacetin-d3
Übersicht
Beschreibung
Phenacetin-d3 (N-(4-[1,1,2-²H₃]ethoxyphenyl)acetamide) is a deuterated analog of Phenacetin (C₁₀H₁₃NO₂), a historically significant analgesic and antipyretic. The incorporation of three deuterium atoms at the ethyl group of the ethoxy substituent enhances its utility in pharmacokinetic and metabolic studies. Deuterated compounds like this compound are widely employed as internal standards in mass spectrometry (MS) due to their near-identical chemical behavior to non-deuterated counterparts, coupled with distinct isotopic signatures .
Vorbereitungsmethoden
Synthetic Routes and Reaction Conditions
The synthesis of Phenacetin-d3 typically involves the deuteration of phenacetin. This process can be achieved through the following steps:
Starting Material: Phenacetin is used as the starting material.
Deuteration: The hydrogen atoms in the acetamide group are replaced with deuterium atoms using deuterated reagents such as deuterated acetic anhydride or deuterated acetic acid.
Reaction Conditions: The reaction is carried out under controlled conditions, often involving a catalyst and specific temperature and pressure settings to ensure complete deuteration.
Industrial Production Methods
Industrial production of this compound follows similar synthetic routes but on a larger scale. The process involves:
Bulk Deuteration: Using large quantities of deuterated reagents.
Purification: The product is purified using techniques such as recrystallization or chromatography to ensure high purity and yield.
Analyse Chemischer Reaktionen
Types of Reactions
Phenacetin-d3 undergoes various chemical reactions, including:
Oxidation: The compound can be oxidized to form corresponding N-oxide derivatives.
Reduction: Reduction reactions can convert the acetamide group to an amine group.
Substitution: The ethoxy group can be substituted with other functional groups under appropriate conditions.
Common Reagents and Conditions
Oxidation: Common oxidizing agents include hydrogen peroxide and peracids.
Reduction: Reducing agents such as lithium aluminum hydride (LiAlH4) or sodium borohydride (NaBH4) are used.
Substitution: Substitution reactions often involve nucleophiles such as halides or amines.
Major Products Formed
Oxidation: N-oxide derivatives.
Reduction: Amine derivatives.
Substitution: Various substituted phenylacetamides.
Wissenschaftliche Forschungsanwendungen
Pharmacokinetics and Metabolism Studies
Phenacetin-d3 is often employed in studies aimed at understanding the metabolic pathways of phenacetin. The incorporation of deuterium allows for the differentiation between the parent compound and its metabolites during analytical measurements.
Key Findings
- Metabolic Pathways : Research indicates that phenacetin undergoes extensive hepatic metabolism, primarily via O-dealkylation to form paracetamol (acetaminophen) and N-hydroxylation to form N-hydroxyphenacetin. The use of this compound facilitates the tracing of these metabolic processes due to its distinct isotopic signature .
- Bioavailability Studies : this compound is used as a standard in bioavailability studies to assess the absorption and distribution of phenacetin in biological systems. These studies provide insights into the drug's efficacy and safety profiles.
Toxicological Assessments
Phenacetin has been associated with various adverse health effects, including nephrotoxicity and carcinogenicity. This compound plays a crucial role in toxicological assessments by allowing researchers to track the compound’s effects on biological systems without interference from non-deuterated forms.
Case Studies
- Renal Toxicity : A study involving rats demonstrated that administration of phenacetin led to significant renal damage characterized by hyperplasia and neoplasia in renal tissues. The use of this compound provided clarity on the specific pathways leading to these toxic effects, as it helped in quantifying the levels of metabolites formed in renal tissues .
- Carcinogenicity Research : Investigations into the carcinogenic potential of phenacetin have utilized this compound to elucidate mechanisms of DNA damage and chromosomal aberrations induced by phenacetin exposure. The isotopic labeling allowed for precise measurements of DNA adducts formed during metabolic activation .
Cancer Research Applications
This compound has been employed in cancer research to study its effects on various cancer cell lines, including melanoma and bladder cancer cells.
Research Highlights
- Melanoma Studies : A recent study explored the effects of low-diluted phenacetin on murine melanoma cells, showing that it disrupted cell proliferation within a fibronectin matrix environment. The incorporation of deuterated compounds helped clarify the mechanisms involved in these cellular responses .
- Bladder Cancer Risk Assessment : Epidemiological studies have linked heavy use of phenacetin-containing analgesics with increased risks of bladder cancer. This compound has been used in laboratory settings to simulate human exposure and assess genetic damage mechanisms involved in tumorigenesis .
Analytical Chemistry Applications
In analytical chemistry, this compound serves as an internal standard for quantifying phenacetin concentrations in biological samples using techniques such as mass spectrometry (MS) and high-performance liquid chromatography (HPLC).
Analytical Techniques
- Mass Spectrometry : The unique mass signature of this compound allows for accurate quantification amidst complex biological matrices, enhancing the reliability of pharmacokinetic studies.
- HPLC Method Development : Researchers have developed HPLC methods utilizing this compound as a standard to improve the resolution and sensitivity of detecting phenacetin and its metabolites in plasma and urine samples.
Wirkmechanismus
The mechanism of action of Phenacetin-d3 involves its interaction with biological targets similar to phenacetin. The deuterium atoms can influence the compound’s metabolic stability, leading to slower metabolism and prolonged activity. The molecular targets and pathways involved include:
Enzymatic Interaction: Interaction with enzymes involved in drug metabolism, such as cytochrome P450 enzymes.
Pharmacokinetics: Altered absorption, distribution, metabolism, and excretion (ADME) profiles due to deuterium substitution.
Vergleich Mit ähnlichen Verbindungen
Molecular Properties
Table 1: Molecular Characteristics of Phenacetin and Its Deuterated Analogs
Compound | Molecular Formula | Molecular Weight | Deuterium Substitution Sites |
---|---|---|---|
Phenacetin | C₁₀H₁₃NO₂ | 179.22 g/mol | None |
Phenacetin-d1 | C₁₀H₁₂DNO₂ | 180.22 g/mol | One hydrogen in the ethoxy ethyl group |
Phenacetin-d3 | C₁₀H₁₀D₃NO₂ | 182.23 g/mol | Three hydrogens in the ethoxy ethyl group |
Key Notes:
- This compound exhibits a 3.01 g/mol increase over non-deuterated Phenacetin, enabling unambiguous differentiation in MS .
- Substitution sites are critical for metabolic stability. Deuterium in the ethoxy group slows O-deethylation, a primary metabolic pathway .
Analytical Data
Table 2: Mass Spectrometry Comparison
Note: this compound’s fragmentation mirrors Phenacetin but shifted by +3 m/z due to deuterium. For example, the loss of -C₂H₅O (45 Da) becomes -C₂D₃H₂O (48 Da), altering fragment masses .
Biologische Aktivität
Phenacetin-d3, a deuterated derivative of phenacetin, is utilized in various biological and pharmacological studies due to its unique isotopic labeling, which aids in understanding drug metabolism and pharmacokinetics. This article explores the biological activity of this compound, including its mechanisms of action, metabolic pathways, and implications in research.
Overview of this compound
This compound (chemical formula: CHDNO$$) is a stable isotope-labeled compound that serves as a valuable tool in pharmacological research. The incorporation of deuterium atoms enhances the stability and metabolic profile of the compound compared to its non-deuterated counterpart.
The mechanism of action of this compound is akin to that of phenacetin, primarily involving its analgesic and antipyretic effects. The deuterium substitution influences the compound's metabolic stability, resulting in:
- Slower Metabolism : The presence of deuterium atoms leads to reduced metabolic rates, prolonging the compound's activity in biological systems.
- Enzymatic Interaction : this compound interacts with cytochrome P450 enzymes, which are crucial for drug metabolism. This interaction alters the absorption, distribution, metabolism, and excretion (ADME) profiles of the drug .
Metabolic Pathways
This compound undergoes several metabolic transformations:
- Oxidative De-ethylation : This is the primary metabolic pathway leading to the formation of N-acetyl-p-aminophenol (paracetamol), which is the active analgesic metabolite.
- N-Deacetylation : Produces p-phenetidine, a metabolite associated with potential carcinogenic effects.
- Hydroxylation : Involves ring modifications leading to various hydroxylated metabolites .
The following table summarizes key metabolic pathways for this compound:
Metabolic Pathway | Primary Product | Enzyme Involvement |
---|---|---|
Oxidative De-ethylation | N-acetyl-p-aminophenol | Cytochrome P450 |
N-Deacetylation | p-Phenetidine | Various hepatic enzymes |
Hydroxylation | Hydroxyphenacetin | Cytochrome P450 |
Biological Activity and Toxicity Studies
Research has shown that phenacetin and its derivatives can induce cytotoxicity and have implications for carcinogenesis. A notable study demonstrated that both phenacetin and acetaminophen could induce morphological transformation in cultured mouse embryo cells at certain concentrations. Specifically, phenacetin was found to be 1.1 to 3.0 times more potent than acetaminophen in inducing atypical foci .
Case Studies
- Renal Cancer Risk : Epidemiological studies have linked long-term use of phenacetin-containing analgesics with an increased risk of renal cancer. A case-control study indicated that heavy users had a significant risk increase for bladder cancer .
- Micronucleus Induction : In animal models, phenacetin has been shown to increase the frequency of micronuclei in bone marrow cells, indicating potential genotoxic effects. This was observed after both oral and intraperitoneal administration .
Research Applications
This compound's unique properties make it suitable for various research applications:
Q & A
Basic Research Questions
Q. What are the validated analytical methods for quantifying Phenacetin-d3 in biological matrices, and how do they address isotopic interference?
- Methodological Answer : High-performance liquid chromatography-tandem mass spectrometry (HPLC-MS/MS) is the gold standard. Deuterated analogs like this compound introduce isotopic peaks, which must be resolved using optimized chromatographic conditions (e.g., C18 columns with gradient elution). Calibration curves should include matrix-matched standards to correct for ion suppression/enhancement .
Q. How is the isotopic purity of this compound confirmed, and what thresholds are acceptable for pharmacokinetic studies?
- Methodological Answer : Isotopic purity is assessed via nuclear magnetic resonance (NMR) spectroscopy and mass spectrometry. A minimum isotopic enrichment of 98% is required to minimize interference from non-deuterated species. For instance, -NMR can detect residual protons at the deuterated acetophenone moiety, while LC-MS quantifies the isotope pattern .
Q. What synthetic routes are employed for this compound, and how do they ensure regioselective deuterium incorporation?
- Methodological Answer : this compound is synthesized via acid-catalyzed deuterium exchange using DO or deuterated acetic anhydride. Regioselectivity at the ethyl group is confirmed by -NMR. Alternative routes involve Pd-catalyzed deuteration of precursor alkenes, with yields >85% reported in anhydrous conditions .
Advanced Research Questions
Q. How do metabolic pathways of this compound differ from its non-deuterated counterpart in in vitro hepatocyte models?
- Methodological Answer : Comparative metabolism studies require incubation with human hepatocytes and UPLC-QTOF-MS analysis. Deuterated analogs may exhibit kinetic isotope effects (KIE), slowing CYP1A2-mediated N-deethylation. Data normalization to protein content and statistical validation (e.g., ANOVA with post-hoc tests) are critical to confirm KIE significance .
Q. What experimental designs mitigate batch-to-batch variability in this compound pharmacokinetic studies?
- Methodological Answer : Use a crossover design with internal standardization (e.g., Phenacetin-) to control for inter-subject variability. Power analysis should determine sample size (n ≥ 6 for rodent studies). Batch effects are minimized via randomization and blinding during dosing and sample analysis .
Q. How can contradictory data on this compound nephrotoxicity be resolved across in vivo models?
- Methodological Answer : Contradictions often arise from species-specific CYP450 expression. A tiered approach is recommended:
Meta-analysis of existing data to identify confounding variables (e.g., dosing regimens).
Mechanistic studies using CRISPR-engineered murine models with humanized CYP1A3.
Omics integration (transcriptomics + metabolomics) to map toxicity pathways .
Q. Methodological Best Practices
- Isotopic Interference : Always include a solvent blank and deuterium-free controls to distinguish analyte signals from background noise .
- Statistical Rigor : Report effect sizes (Cohen’s d) alongside p-values to contextualize findings .
- Ethical Compliance : Adhere to OECD Guidelines for chemical safety testing, particularly for nephrotoxicity assays .
Eigenschaften
IUPAC Name |
2,2,2-trideuterio-N-(4-ethoxyphenyl)acetamide | |
---|---|---|
Source | PubChem | |
URL | https://pubchem.ncbi.nlm.nih.gov | |
Description | Data deposited in or computed by PubChem | |
InChI |
InChI=1S/C10H13NO2/c1-3-13-10-6-4-9(5-7-10)11-8(2)12/h4-7H,3H2,1-2H3,(H,11,12)/i2D3 | |
Source | PubChem | |
URL | https://pubchem.ncbi.nlm.nih.gov | |
Description | Data deposited in or computed by PubChem | |
InChI Key |
CPJSUEIXXCENMM-BMSJAHLVSA-N | |
Source | PubChem | |
URL | https://pubchem.ncbi.nlm.nih.gov | |
Description | Data deposited in or computed by PubChem | |
Canonical SMILES |
CCOC1=CC=C(C=C1)NC(=O)C | |
Source | PubChem | |
URL | https://pubchem.ncbi.nlm.nih.gov | |
Description | Data deposited in or computed by PubChem | |
Isomeric SMILES |
[2H]C([2H])([2H])C(=O)NC1=CC=C(C=C1)OCC | |
Source | PubChem | |
URL | https://pubchem.ncbi.nlm.nih.gov | |
Description | Data deposited in or computed by PubChem | |
Molecular Formula |
C10H13NO2 | |
Source | PubChem | |
URL | https://pubchem.ncbi.nlm.nih.gov | |
Description | Data deposited in or computed by PubChem | |
Molecular Weight |
182.23 g/mol | |
Source | PubChem | |
URL | https://pubchem.ncbi.nlm.nih.gov | |
Description | Data deposited in or computed by PubChem | |
Haftungsausschluss und Informationen zu In-Vitro-Forschungsprodukten
Bitte beachten Sie, dass alle Artikel und Produktinformationen, die auf BenchChem präsentiert werden, ausschließlich zu Informationszwecken bestimmt sind. Die auf BenchChem zum Kauf angebotenen Produkte sind speziell für In-vitro-Studien konzipiert, die außerhalb lebender Organismen durchgeführt werden. In-vitro-Studien, abgeleitet von dem lateinischen Begriff "in Glas", beinhalten Experimente, die in kontrollierten Laborumgebungen unter Verwendung von Zellen oder Geweben durchgeführt werden. Es ist wichtig zu beachten, dass diese Produkte nicht als Arzneimittel oder Medikamente eingestuft sind und keine Zulassung der FDA für die Vorbeugung, Behandlung oder Heilung von medizinischen Zuständen, Beschwerden oder Krankheiten erhalten haben. Wir müssen betonen, dass jede Form der körperlichen Einführung dieser Produkte in Menschen oder Tiere gesetzlich strikt untersagt ist. Es ist unerlässlich, sich an diese Richtlinien zu halten, um die Einhaltung rechtlicher und ethischer Standards in Forschung und Experiment zu gewährleisten.