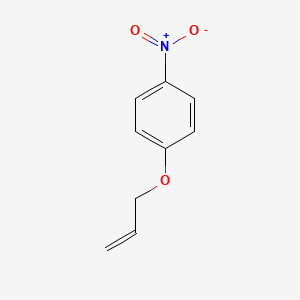
Allyl p-nitrophenyl ether
Übersicht
Beschreibung
Allyl p-nitrophenyl ether is an organic compound characterized by the presence of an allyl group and a p-nitrophenyl group connected through an ether linkage
Vorbereitungsmethoden
Synthetic Routes and Reaction Conditions: Allyl p-nitrophenyl ether can be synthesized through several methods, including the Williamson ether synthesis, which involves the reaction of an alkoxide ion with an alkyl halide . Another method involves the Claisen rearrangement, where this compound undergoes a thermal rearrangement to form the desired product .
Industrial Production Methods: Industrial production of this compound typically involves large-scale Williamson ether synthesis due to its efficiency and scalability. The reaction is carried out under controlled conditions to ensure high yield and purity of the product.
Analyse Chemischer Reaktionen
Types of Reactions: Allyl p-nitrophenyl ether undergoes various chemical reactions, including:
Oxidation: The compound can be oxidized to form corresponding aldehydes or acids.
Reduction: Reduction reactions can convert the nitro group to an amine group.
Substitution: Nucleophilic substitution reactions can replace the nitro group with other functional groups.
Common Reagents and Conditions:
Oxidation: Common oxidizing agents include potassium permanganate and chromium trioxide.
Reduction: Reducing agents such as hydrogen gas with a palladium catalyst or lithium aluminum hydride are used.
Substitution: Reagents like sodium hydroxide or other nucleophiles are employed under basic conditions.
Major Products Formed:
Oxidation: Formation of p-nitrobenzaldehyde or p-nitrobenzoic acid.
Reduction: Formation of p-aminophenyl ether.
Substitution: Formation of various substituted phenyl ethers depending on the nucleophile used.
Wissenschaftliche Forschungsanwendungen
Chemical Research Applications
1. Microwave-Assisted Organic Synthesis
Allyl p-nitrophenyl ether is widely used as a model compound in microwave-assisted organic synthesis. Studies have demonstrated that this compound exhibits significant rate enhancements in reactions like the unimolecular Claisen rearrangement when subjected to microwave heating compared to conventional heating methods. For instance, a study reported a four-fold increase in reaction rate under microwave conditions, attributed to selective heating effects that lead to localized "hot spots" around the reactant molecules .
2. Claisen Rearrangement Studies
The Claisen rearrangement involving this compound serves as a critical mechanism for understanding reaction kinetics and mechanisms in organic chemistry. The compound's ability to undergo this rearrangement allows researchers to explore fundamental aspects of organic reaction pathways and their temperature dependencies .
Biological Applications
1. Antiproliferative Effects
Research has indicated that this compound exhibits antiproliferative activity against various cancer cell lines, particularly breast cancer cells (MCF-7). In vitro studies have shown that the compound can influence cell viability and proliferation, making it a candidate for further investigation in cancer therapeutics .
2. Interaction with Biomolecules
The compound's interactions with biomolecules have been explored, particularly regarding its potential biological activities. These interactions can provide insights into its effects on cellular processes, gene expression, and metabolic pathways, which are crucial for understanding its pharmacological potential.
Medicinal Chemistry Applications
1. Drug Development
This compound is being investigated as a precursor for synthesizing pharmacologically active compounds. Its structural features allow for modifications that can lead to the development of new drugs targeting various diseases. The compound's reactivity makes it valuable in synthesizing complex organic molecules that could serve therapeutic purposes.
2. Protective Group in Organic Synthesis
In synthetic organic chemistry, this compound can function as a protective group for alcohols during multi-step synthesis processes. Its stability under various reaction conditions allows chemists to manipulate other functional groups without affecting the ether linkage .
Industrial Applications
1. Synthesis of Specialty Chemicals
In industrial settings, this compound is utilized in synthesizing specialty chemicals and materials. Its versatility in chemical reactions makes it suitable for producing compounds used in various applications, including polymers and agrochemicals .
2. Large-Scale Production Methods
The industrial production of this compound typically employs methods like Williamson ether synthesis due to their efficiency and scalability. This process involves the reaction of an alkoxide ion with an alkyl halide under controlled conditions to ensure high yield and purity .
Case Studies
Wirkmechanismus
The mechanism of action of allyl p-nitrophenyl ether primarily involves its reactivity under microwave irradiation. The compound absorbs microwave energy, leading to localized heating and accelerated reaction rates. This selective heating effect enhances the efficiency of various chemical reactions, such as the Claisen rearrangement . The molecular targets and pathways involved include the activation of the ether linkage and the nitro group, facilitating rearrangement and substitution reactions .
Vergleich Mit ähnlichen Verbindungen
4-Nitroanisole: Similar in structure but with a methoxy group instead of an allyl group.
p-Nitrophenyl acetate: Contains an ester linkage instead of an ether linkage.
p-Nitrophenyl ether: Lacks the allyl group, making it less reactive in certain conditions.
Uniqueness: Allyl p-nitrophenyl ether is unique due to its combination of an allyl group and a p-nitrophenyl group, which imparts distinct reactivity and makes it a valuable compound for studying microwave-assisted reactions and other advanced synthetic methodologies .
Biologische Aktivität
Allyl p-nitrophenyl ether (ApNE) is a compound that has garnered attention in the field of medicinal chemistry due to its potential biological activities, particularly in antimicrobial and anticancer applications. This article presents a comprehensive overview of the biological activity of ApNE, including its mechanisms of action, experimental findings, and relevant case studies.
Chemical Structure and Properties
This compound is characterized by the presence of an allyl group attached to a p-nitrophenyl moiety. The molecular structure can be represented as follows:
This structure contributes to its unique chemical properties, including its reactivity and interaction with biological targets.
The biological activity of ApNE is primarily attributed to its ability to interact with various molecular targets. Research indicates that ApNE may influence several cellular pathways:
- Antimicrobial Activity : ApNE has shown effectiveness against various strains of bacteria, including both Gram-positive and Gram-negative species. Its mechanism involves disrupting bacterial cell membranes and inhibiting essential enzymatic functions.
- Anticancer Properties : Studies have demonstrated that ApNE can induce apoptosis in cancer cells by modulating pathways associated with oxidative stress and inflammation. It interacts with tubulin, disrupting microtubule dynamics, which is crucial for cell division.
Antimicrobial Activity
A study evaluated the minimum inhibitory concentration (MIC) of ApNE against several bacterial strains. The results are summarized in Table 1:
Bacterial Strain | MIC (mM) |
---|---|
E. coli | 6.3 |
S. aureus | 5.0 |
P. aeruginosa | 4.5 |
E. faecalis | 7.0 |
These findings suggest that ApNE exhibits significant antimicrobial activity, making it a candidate for further development as an antimicrobial agent .
Anticancer Activity
In vitro studies have assessed the antiproliferative effects of ApNE on various cancer cell lines, particularly breast cancer cells (MCF-7). The results indicated that ApNE has an IC50 value comparable to established anticancer agents, demonstrating its potential as a therapeutic compound.
Table 2 summarizes the antiproliferative activity of ApNE:
Compound | Cell Line | IC50 (µM) |
---|---|---|
This compound | MCF-7 | 12 |
CA-4 (reference) | MCF-7 | 3.9 |
The data indicate that while ApNE is less potent than CA-4, it still possesses significant antiproliferative properties .
Case Studies
- Microwave-Assisted Synthesis : A study investigated the microwave-assisted synthesis of ApNE and its implications for enhancing reaction rates in organic synthesis. The findings demonstrated a four-fold increase in reaction rates under microwave conditions compared to conventional heating methods .
- Photodegradable Antimicrobial Agents : Research explored the photodegradation of compounds related to ApNE, revealing that under UV light exposure, degradation products retained antimicrobial activity against resistant bacterial strains .
Eigenschaften
IUPAC Name |
1-nitro-4-prop-2-enoxybenzene | |
---|---|---|
Source | PubChem | |
URL | https://pubchem.ncbi.nlm.nih.gov | |
Description | Data deposited in or computed by PubChem | |
InChI |
InChI=1S/C9H9NO3/c1-2-7-13-9-5-3-8(4-6-9)10(11)12/h2-6H,1,7H2 | |
Source | PubChem | |
URL | https://pubchem.ncbi.nlm.nih.gov | |
Description | Data deposited in or computed by PubChem | |
InChI Key |
DJOGGVVIZHGION-UHFFFAOYSA-N | |
Source | PubChem | |
URL | https://pubchem.ncbi.nlm.nih.gov | |
Description | Data deposited in or computed by PubChem | |
Canonical SMILES |
C=CCOC1=CC=C(C=C1)[N+](=O)[O-] | |
Source | PubChem | |
URL | https://pubchem.ncbi.nlm.nih.gov | |
Description | Data deposited in or computed by PubChem | |
Molecular Formula |
C9H9NO3 | |
Source | PubChem | |
URL | https://pubchem.ncbi.nlm.nih.gov | |
Description | Data deposited in or computed by PubChem | |
DSSTOX Substance ID |
DTXSID50277447 | |
Record name | Allyl p-nitrophenyl ether | |
Source | EPA DSSTox | |
URL | https://comptox.epa.gov/dashboard/DTXSID50277447 | |
Description | DSSTox provides a high quality public chemistry resource for supporting improved predictive toxicology. | |
Molecular Weight |
179.17 g/mol | |
Source | PubChem | |
URL | https://pubchem.ncbi.nlm.nih.gov | |
Description | Data deposited in or computed by PubChem | |
CAS No. |
1568-66-7 | |
Record name | 1-Nitro-4-(2-propen-1-yloxy)benzene | |
Source | CAS Common Chemistry | |
URL | https://commonchemistry.cas.org/detail?cas_rn=1568-66-7 | |
Description | CAS Common Chemistry is an open community resource for accessing chemical information. Nearly 500,000 chemical substances from CAS REGISTRY cover areas of community interest, including common and frequently regulated chemicals, and those relevant to high school and undergraduate chemistry classes. This chemical information, curated by our expert scientists, is provided in alignment with our mission as a division of the American Chemical Society. | |
Explanation | The data from CAS Common Chemistry is provided under a CC-BY-NC 4.0 license, unless otherwise stated. | |
Record name | NSC 2375 | |
Source | ChemIDplus | |
URL | https://pubchem.ncbi.nlm.nih.gov/substance/?source=chemidplus&sourceid=0001568667 | |
Description | ChemIDplus is a free, web search system that provides access to the structure and nomenclature authority files used for the identification of chemical substances cited in National Library of Medicine (NLM) databases, including the TOXNET system. | |
Record name | Allyl p-nitrophenyl ether | |
Source | DTP/NCI | |
URL | https://dtp.cancer.gov/dtpstandard/servlet/dwindex?searchtype=NSC&outputformat=html&searchlist=2375 | |
Description | The NCI Development Therapeutics Program (DTP) provides services and resources to the academic and private-sector research communities worldwide to facilitate the discovery and development of new cancer therapeutic agents. | |
Explanation | Unless otherwise indicated, all text within NCI products is free of copyright and may be reused without our permission. Credit the National Cancer Institute as the source. | |
Record name | Allyl p-nitrophenyl ether | |
Source | EPA DSSTox | |
URL | https://comptox.epa.gov/dashboard/DTXSID50277447 | |
Description | DSSTox provides a high quality public chemistry resource for supporting improved predictive toxicology. | |
Synthesis routes and methods
Procedure details
Retrosynthesis Analysis
AI-Powered Synthesis Planning: Our tool employs the Template_relevance Pistachio, Template_relevance Bkms_metabolic, Template_relevance Pistachio_ringbreaker, Template_relevance Reaxys, Template_relevance Reaxys_biocatalysis model, leveraging a vast database of chemical reactions to predict feasible synthetic routes.
One-Step Synthesis Focus: Specifically designed for one-step synthesis, it provides concise and direct routes for your target compounds, streamlining the synthesis process.
Accurate Predictions: Utilizing the extensive PISTACHIO, BKMS_METABOLIC, PISTACHIO_RINGBREAKER, REAXYS, REAXYS_BIOCATALYSIS database, our tool offers high-accuracy predictions, reflecting the latest in chemical research and data.
Strategy Settings
Precursor scoring | Relevance Heuristic |
---|---|
Min. plausibility | 0.01 |
Model | Template_relevance |
Template Set | Pistachio/Bkms_metabolic/Pistachio_ringbreaker/Reaxys/Reaxys_biocatalysis |
Top-N result to add to graph | 6 |
Feasible Synthetic Routes
Q1: How does Allyl p-nitrophenyl ether behave differently under microwave heating compared to conventional heating, and what causes this difference?
A1: Research has shown that this compound, when dissolved in a non-absorbing solvent like naphthalene and subjected to microwave irradiation, can exhibit significant rate enhancements in its unimolecular Claisen rearrangement reaction compared to conventional heating methods []. This rate enhancement is attributed to the selective heating of this compound, a microwave-absorbing reactant, in the non-absorbing solvent. This selective heating leads to localized "hot spots" around the this compound molecules, resulting in a higher effective temperature experienced by the reactant compared to the measured bulk temperature [].
Haftungsausschluss und Informationen zu In-Vitro-Forschungsprodukten
Bitte beachten Sie, dass alle Artikel und Produktinformationen, die auf BenchChem präsentiert werden, ausschließlich zu Informationszwecken bestimmt sind. Die auf BenchChem zum Kauf angebotenen Produkte sind speziell für In-vitro-Studien konzipiert, die außerhalb lebender Organismen durchgeführt werden. In-vitro-Studien, abgeleitet von dem lateinischen Begriff "in Glas", beinhalten Experimente, die in kontrollierten Laborumgebungen unter Verwendung von Zellen oder Geweben durchgeführt werden. Es ist wichtig zu beachten, dass diese Produkte nicht als Arzneimittel oder Medikamente eingestuft sind und keine Zulassung der FDA für die Vorbeugung, Behandlung oder Heilung von medizinischen Zuständen, Beschwerden oder Krankheiten erhalten haben. Wir müssen betonen, dass jede Form der körperlichen Einführung dieser Produkte in Menschen oder Tiere gesetzlich strikt untersagt ist. Es ist unerlässlich, sich an diese Richtlinien zu halten, um die Einhaltung rechtlicher und ethischer Standards in Forschung und Experiment zu gewährleisten.