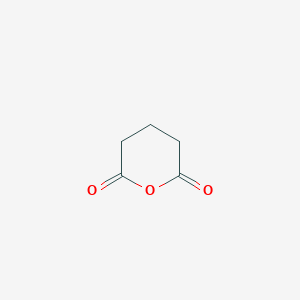
Glutaric anhydride
Übersicht
Beschreibung
Glutaric anhydride is a cyclic anhydride derived from glutaric acid. It features a five-membered ring structure with two carbonyl groups, making it a versatile compound in organic synthesis. This compound is widely used in the production of various polymers, resins, and pharmaceuticals due to its reactivity as an electrophile .
Wirkmechanismus
Target of Action
Glutaric anhydride primarily targets enzymes such as Aspartate aminotransferase, Branched-chain-amino-acid aminotransferase, and Glutamate decarboxylase alpha in Escherichia coli . These enzymes play crucial roles in various biochemical processes, including amino acid metabolism.
Mode of Action
this compound interacts with its targets through a process known as nucleophilic acyl substitution . During this reaction, the leaving group is removed from the anhydride, and the neutral nucleophile loses a hydrogen . This interaction results in the formation of a new acyl compound .
Biochemical Pathways
this compound affects several biochemical pathways. It is produced in the body during the metabolism of various amino acids, such as lysine and tryptophan . Four distinct pathways have been used for the bioproduction of glutaric acid: the α-ketoglutarate reduction method, the α-ketoglutarate carbon chain extension and decarboxylation process, the reverse adipate degradation pathway, and the l-lysine catabolism pathway .
Pharmacokinetics
It is known that this compound readily hydrolyzes to form glutaric acid .
Result of Action
The action of this compound at the molecular and cellular level results in several effects. It disrupts neurotransmission, redox homeostasis, bioenergetics, and myelination, and induces reactive astrogliosis and neuronal death . Moreover, this compound enhances the properties of copolymers, particularly in terms of shape memory and self-healing capabilities .
Action Environment
Environmental factors can influence the action of this compound. For instance, the presence of water can trigger the hydrolysis of this compound to glutaric acid . Additionally, the rate of hydrolysis and hence of pH change can be used to control the rate of gel formation .
Biochemische Analyse
Biochemical Properties
Glutaric anhydride plays a significant role in biochemical reactions. It has been used in the modification of cellulose surfaces, introducing carboxylate functional groups that can increase the hydrophilicity of cellulose fibers and water dispersibility . It also enhances the properties of copolymers, particularly in terms of shape memory and self-healing capabilities .
Cellular Effects
In the context of cellular effects, this compound has been shown to influence the performance of lithium-ion cells. It forms a homogeneous fluorophosphates-containing interphase, lowering the formation of LiF, a highly resistive species . This suggests that this compound can influence cell function by modifying the cell’s electrochemical properties.
Molecular Mechanism
The molecular mechanism of this compound involves its reaction with other molecules to form new compounds. For instance, it has been used in the esterification of bagasse, a byproduct of sugarcane, where it reacts with the hydroxyl groups in bagasse to introduce glutaryl groups . This indicates that this compound can interact with biomolecules, leading to changes in their structure and function.
Temporal Effects in Laboratory Settings
In laboratory settings, this compound has been shown to have temporal effects on lithium-ion cells. The addition of this compound to the electrolyte of these cells resulted in superior cycling performance compared to the baseline electrolyte . This suggests that this compound can have long-term effects on cellular function in in vitro studies.
Metabolic Pathways
This compound is involved in several metabolic pathways. For instance, it has been used in the α-ketoglutarate reduction method, the α-ketoglutarate carbon chain extension and decarboxylation process, and the l-lysine catabolism pathway for the bioproduction of glutaric acid .
Vorbereitungsmethoden
Synthetic Routes and Reaction Conditions: Glutaric anhydride is typically synthesized by the dehydration of glutaric acid. This process involves heating glutaric acid to remove water, resulting in the formation of the cyclic anhydride . The reaction can be represented as follows:
C5H8O4→C5H6O3+H2O
Industrial Production Methods: In industrial settings, this compound is produced using similar dehydration methods but on a larger scale. The process often involves the use of catalysts to enhance the reaction rate and yield. The anhydride is then purified through distillation or recrystallization .
Types of Reactions:
Nucleophilic Substitution: this compound undergoes nucleophilic attack due to its electrophilic carbonyl groups.
Hydrolysis: When exposed to water, this compound hydrolyzes to form glutaric acid.
Reduction: It can be reduced to form primary alcohols using hydride reagents.
Common Reagents and Conditions:
Amines: React with this compound to form amides.
Alcohols: React to form esters.
Water: Causes hydrolysis to glutaric acid.
Hydride Reagents: Used for reduction reactions.
Major Products:
Amides: Formed from reactions with amines.
Esters: Formed from reactions with alcohols.
Glutaric Acid: Formed from hydrolysis.
Wissenschaftliche Forschungsanwendungen
Glutaric anhydride has a wide range of applications in scientific research:
Chemistry: Used as a building block in organic synthesis and polymer chemistry.
Biology: Employed in bioconjugation techniques for modifying biomolecules.
Medicine: Utilized in drug design and development due to its ability to form stable intermediates.
Industry: Used in the production of polymers, corrosion inhibitors, and surfactants.
Vergleich Mit ähnlichen Verbindungen
Succinic Anhydride: Another cyclic anhydride with similar reactivity but a four-membered ring structure.
Adipic Anhydride: Features a six-membered ring and is used in similar applications.
Uniqueness: Glutaric anhydride’s five-membered ring structure provides a balance between reactivity and stability, making it particularly useful in polymer chemistry and drug design. Its ability to form stable intermediates and enhance molecular interactions sets it apart from other anhydrides .
Eigenschaften
IUPAC Name |
oxane-2,6-dione | |
---|---|---|
Source | PubChem | |
URL | https://pubchem.ncbi.nlm.nih.gov | |
Description | Data deposited in or computed by PubChem | |
InChI |
InChI=1S/C5H6O3/c6-4-2-1-3-5(7)8-4/h1-3H2 | |
Source | PubChem | |
URL | https://pubchem.ncbi.nlm.nih.gov | |
Description | Data deposited in or computed by PubChem | |
InChI Key |
VANNPISTIUFMLH-UHFFFAOYSA-N | |
Source | PubChem | |
URL | https://pubchem.ncbi.nlm.nih.gov | |
Description | Data deposited in or computed by PubChem | |
Canonical SMILES |
C1CC(=O)OC(=O)C1 | |
Source | PubChem | |
URL | https://pubchem.ncbi.nlm.nih.gov | |
Description | Data deposited in or computed by PubChem | |
Molecular Formula |
C5H6O3 | |
Source | PubChem | |
URL | https://pubchem.ncbi.nlm.nih.gov | |
Description | Data deposited in or computed by PubChem | |
DSSTOX Substance ID |
DTXSID5044362 | |
Record name | Glutaric anhydride | |
Source | EPA DSSTox | |
URL | https://comptox.epa.gov/dashboard/DTXSID5044362 | |
Description | DSSTox provides a high quality public chemistry resource for supporting improved predictive toxicology. | |
Molecular Weight |
114.10 g/mol | |
Source | PubChem | |
URL | https://pubchem.ncbi.nlm.nih.gov | |
Description | Data deposited in or computed by PubChem | |
Physical Description |
Off-white flakes or crystals; [Alfa Aesar MSDS] | |
Record name | Glutaric anhydride | |
Source | Haz-Map, Information on Hazardous Chemicals and Occupational Diseases | |
URL | https://haz-map.com/Agents/20962 | |
Description | Haz-Map® is an occupational health database designed for health and safety professionals and for consumers seeking information about the adverse effects of workplace exposures to chemical and biological agents. | |
Explanation | Copyright (c) 2022 Haz-Map(R). All rights reserved. Unless otherwise indicated, all materials from Haz-Map are copyrighted by Haz-Map(R). No part of these materials, either text or image may be used for any purpose other than for personal use. Therefore, reproduction, modification, storage in a retrieval system or retransmission, in any form or by any means, electronic, mechanical or otherwise, for reasons other than personal use, is strictly prohibited without prior written permission. | |
CAS No. |
108-55-4 | |
Record name | Glutaric anhydride | |
Source | CAS Common Chemistry | |
URL | https://commonchemistry.cas.org/detail?cas_rn=108-55-4 | |
Description | CAS Common Chemistry is an open community resource for accessing chemical information. Nearly 500,000 chemical substances from CAS REGISTRY cover areas of community interest, including common and frequently regulated chemicals, and those relevant to high school and undergraduate chemistry classes. This chemical information, curated by our expert scientists, is provided in alignment with our mission as a division of the American Chemical Society. | |
Explanation | The data from CAS Common Chemistry is provided under a CC-BY-NC 4.0 license, unless otherwise stated. | |
Record name | Glutaric anhydride | |
Source | ChemIDplus | |
URL | https://pubchem.ncbi.nlm.nih.gov/substance/?source=chemidplus&sourceid=0000108554 | |
Description | ChemIDplus is a free, web search system that provides access to the structure and nomenclature authority files used for the identification of chemical substances cited in National Library of Medicine (NLM) databases, including the TOXNET system. | |
Record name | GLUTARIC ANHYDRIDE | |
Source | DTP/NCI | |
URL | https://dtp.cancer.gov/dtpstandard/servlet/dwindex?searchtype=NSC&outputformat=html&searchlist=16640 | |
Description | The NCI Development Therapeutics Program (DTP) provides services and resources to the academic and private-sector research communities worldwide to facilitate the discovery and development of new cancer therapeutic agents. | |
Explanation | Unless otherwise indicated, all text within NCI products is free of copyright and may be reused without our permission. Credit the National Cancer Institute as the source. | |
Record name | 2H-Pyran-2,6(3H)-dione, dihydro- | |
Source | EPA Chemicals under the TSCA | |
URL | https://www.epa.gov/chemicals-under-tsca | |
Description | EPA Chemicals under the Toxic Substances Control Act (TSCA) collection contains information on chemicals and their regulations under TSCA, including non-confidential content from the TSCA Chemical Substance Inventory and Chemical Data Reporting. | |
Record name | Glutaric anhydride | |
Source | EPA DSSTox | |
URL | https://comptox.epa.gov/dashboard/DTXSID5044362 | |
Description | DSSTox provides a high quality public chemistry resource for supporting improved predictive toxicology. | |
Record name | Glutaric anhydride | |
Source | European Chemicals Agency (ECHA) | |
URL | https://echa.europa.eu/substance-information/-/substanceinfo/100.003.267 | |
Description | The European Chemicals Agency (ECHA) is an agency of the European Union which is the driving force among regulatory authorities in implementing the EU's groundbreaking chemicals legislation for the benefit of human health and the environment as well as for innovation and competitiveness. | |
Explanation | Use of the information, documents and data from the ECHA website is subject to the terms and conditions of this Legal Notice, and subject to other binding limitations provided for under applicable law, the information, documents and data made available on the ECHA website may be reproduced, distributed and/or used, totally or in part, for non-commercial purposes provided that ECHA is acknowledged as the source: "Source: European Chemicals Agency, http://echa.europa.eu/". Such acknowledgement must be included in each copy of the material. ECHA permits and encourages organisations and individuals to create links to the ECHA website under the following cumulative conditions: Links can only be made to webpages that provide a link to the Legal Notice page. | |
Record name | GLUTARIC ANHYDRIDE | |
Source | FDA Global Substance Registration System (GSRS) | |
URL | https://gsrs.ncats.nih.gov/ginas/app/beta/substances/63OFI15S80 | |
Description | The FDA Global Substance Registration System (GSRS) enables the efficient and accurate exchange of information on what substances are in regulated products. Instead of relying on names, which vary across regulatory domains, countries, and regions, the GSRS knowledge base makes it possible for substances to be defined by standardized, scientific descriptions. | |
Explanation | Unless otherwise noted, the contents of the FDA website (www.fda.gov), both text and graphics, are not copyrighted. They are in the public domain and may be republished, reprinted and otherwise used freely by anyone without the need to obtain permission from FDA. Credit to the U.S. Food and Drug Administration as the source is appreciated but not required. | |
Synthesis routes and methods
Procedure details
Retrosynthesis Analysis
AI-Powered Synthesis Planning: Our tool employs the Template_relevance Pistachio, Template_relevance Bkms_metabolic, Template_relevance Pistachio_ringbreaker, Template_relevance Reaxys, Template_relevance Reaxys_biocatalysis model, leveraging a vast database of chemical reactions to predict feasible synthetic routes.
One-Step Synthesis Focus: Specifically designed for one-step synthesis, it provides concise and direct routes for your target compounds, streamlining the synthesis process.
Accurate Predictions: Utilizing the extensive PISTACHIO, BKMS_METABOLIC, PISTACHIO_RINGBREAKER, REAXYS, REAXYS_BIOCATALYSIS database, our tool offers high-accuracy predictions, reflecting the latest in chemical research and data.
Strategy Settings
Precursor scoring | Relevance Heuristic |
---|---|
Min. plausibility | 0.01 |
Model | Template_relevance |
Template Set | Pistachio/Bkms_metabolic/Pistachio_ringbreaker/Reaxys/Reaxys_biocatalysis |
Top-N result to add to graph | 6 |
Feasible Synthetic Routes
ANone: Glutaric anhydride has the molecular formula C5H6O3 and a molecular weight of 114.10 g/mol.
A: this compound has been characterized using various spectroscopic techniques, including Fourier transform infrared (FT-IR) spectroscopy [, , , , , ], carbon-13 nuclear magnetic resonance (13C NMR) spectroscopy [, , , , ], and proton magnetic resonance (1H NMR) spectroscopy [, , , , , ]. These techniques provide information about the functional groups, structure, and dynamics of the molecule. For example, FT-IR spectroscopy confirms the presence of characteristic carbonyl (-C=O) stretching vibrations, while NMR spectroscopy reveals details about the arrangement of carbon and hydrogen atoms within the molecule.
A: this compound is known to react with various functional groups, primarily alcohols and amines. This reactivity forms the basis for its applications in modifying natural fibers [, ], synthesizing polyesters [, , ], and creating crosslinked polymer networks [, ]. For instance, it reacts with hydroxyl groups in cellulose, hemicellulose, and lignin present in bagasse, enhancing its surface adhesion properties for potential biomedical applications [].
A: this compound serves as a versatile building block in organic synthesis, particularly in the formation of five-membered rings like lactones and lactams [, , ]. Its reactivity with nucleophiles, such as Grignard reagents, enables the synthesis of various derivatives, as exemplified by the preparation of 5-decanolide []. Moreover, its use in the Castagnoli-Cushman reaction allows the efficient synthesis of γ- and δ-lactams under mild conditions [, ].
A: The cyclic anhydride structure of this compound imbues it with unique reactivity compared to its linear dicarboxylic acid counterpart. This structure allows for ring-opening reactions with nucleophiles, leading to the formation of various derivatives. The presence of two carbonyl groups provides opportunities for regioselective reactions, as demonstrated in the nickel-catalyzed alkylation of unsymmetrical succinic anhydrides [].
A: Computational studies, particularly density functional theory (DFT) calculations, have provided valuable insights into the conformational preferences, reactivity, and reaction mechanisms involving this compound derivatives [, , , ]. For example, DFT calculations have been employed to rationalize the enantioselectivity observed in the alcoholytic desymmetrization of meso-glutaric anhydrides catalyzed by Cinchona-based sulfonamide catalysts [].
A: Introducing substituents on the this compound ring can significantly influence its reactivity and downstream applications. For instance, incorporating bulky substituents at the 3-position of this compound leads to high diastereoselectivity in the synthesis of γ- and δ-lactams []. Additionally, the presence of electron-withdrawing groups can modulate the reactivity of the anhydride ring, affecting its ring-opening reactions with nucleophiles [].
A: While this compound is inherently sensitive to moisture, its stability can be enhanced by using appropriate storage conditions, such as storing it under anhydrous conditions and in a moisture-free environment. Furthermore, specific formulations can be developed to improve its stability during processing or application. For instance, using non-polar solvents like hexane or toluene during its synthesis can minimize hydrolysis and enhance its shelf life [].
A: Various analytical techniques are employed to characterize and quantify this compound and its derivatives. These include:- Titration: Return-titramatic analysis is a quick and precise method for determining this compound content []. - Chromatographic techniques: High-performance liquid chromatography (HPLC) helps analyze this compound and identify impurities or degradation products [].- Spectroscopic techniques: FT-IR, 1H NMR, and 13C NMR are crucial in characterizing the structure and purity of synthesized compounds [, , , , , , , , , , ]. - Mass spectrometry: Techniques like matrix-assisted laser desorption/ionization time-of-flight mass spectrometry (MALDI-TOF) help determine the molecular weight and analyze oligomers and polymers formed using this compound [, ].- Thermal analysis: Thermogravimetric analysis (TGA) is used to study the thermal stability and decomposition behavior of this compound-based polymers and composites [, ].
Haftungsausschluss und Informationen zu In-Vitro-Forschungsprodukten
Bitte beachten Sie, dass alle Artikel und Produktinformationen, die auf BenchChem präsentiert werden, ausschließlich zu Informationszwecken bestimmt sind. Die auf BenchChem zum Kauf angebotenen Produkte sind speziell für In-vitro-Studien konzipiert, die außerhalb lebender Organismen durchgeführt werden. In-vitro-Studien, abgeleitet von dem lateinischen Begriff "in Glas", beinhalten Experimente, die in kontrollierten Laborumgebungen unter Verwendung von Zellen oder Geweben durchgeführt werden. Es ist wichtig zu beachten, dass diese Produkte nicht als Arzneimittel oder Medikamente eingestuft sind und keine Zulassung der FDA für die Vorbeugung, Behandlung oder Heilung von medizinischen Zuständen, Beschwerden oder Krankheiten erhalten haben. Wir müssen betonen, dass jede Form der körperlichen Einführung dieser Produkte in Menschen oder Tiere gesetzlich strikt untersagt ist. Es ist unerlässlich, sich an diese Richtlinien zu halten, um die Einhaltung rechtlicher und ethischer Standards in Forschung und Experiment zu gewährleisten.