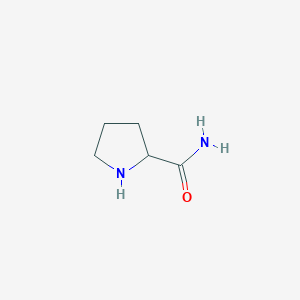
Pyrrolidin-2-carboxamid
Übersicht
Beschreibung
Pyrrolidine-2-carboxamide is a heterocyclic organic compound featuring a five-membered ring containing nitrogen. This compound is of significant interest in medicinal chemistry due to its versatile biological activities and its role as a building block in the synthesis of various bioactive molecules .
Wissenschaftliche Forschungsanwendungen
Pyrrolidine-2-carboxamide has a wide range of applications in scientific research:
Chemistry: It serves as a precursor in the synthesis of complex organic molecules.
Biology: It is used in the study of enzyme inhibitors and receptor modulators.
Medicine: Pyrrolidine-2-carboxamide derivatives are investigated for their potential therapeutic effects, including anti-inflammatory and anticancer activities.
Industry: It is utilized in the production of pharmaceuticals and agrochemicals
Wirkmechanismus
Target of Action
Pyrrolidine-2-carboxamide is a derivative of the pyrrolidine ring, which is a nitrogen heterocycle widely used by medicinal chemists to obtain compounds for the treatment of human diseases Pyrrolidine derivatives have been reported to exhibit activity against various targets, including ck1γ and ck1ε .
Mode of Action
The pyrrolidine ring and its derivatives are known to interact with their targets through various mechanisms, often involving the formation of hydrogen bonds with a variety of enzymes and proteins . This interaction can inhibit the activity of these enzymes and proteins, leading to the therapeutic effects of the compounds .
Biochemical Pathways
Pyrrolidine derivatives have been associated with a wide range of biological activities, including antimicrobial, antiviral, anticancer, anti-inflammatory, anticonvulsant, cholinesterase inhibition, and carbonic anhydrase inhibition . These activities suggest that Pyrrolidine-2-carboxamide may influence multiple biochemical pathways.
Pharmacokinetics
The physicochemical parameters of pyrrolidine and its derivatives have been studied, which could provide insights into their pharmacokinetic properties .
Result of Action
Pyrrolidine derivatives have been reported to exhibit nanomolar activity against certain targets, suggesting potent biological effects . Moreover, some pyrrolidine derivatives have shown potent antitumor activity, suggesting potential applications in cancer therapy .
Action Environment
The stereochemistry of the pyrrolidine ring and the spatial orientation of substituents can lead to different biological profiles of drug candidates, indicating that the molecular environment could influence the compound’s action .
Zukünftige Richtungen
Biochemische Analyse
Biochemical Properties
Pyrrolidine-2-carboxamide has been shown to interact with various enzymes, proteins, and other biomolecules . For instance, it has been reported to exhibit inhibitory activity towards dipeptidyl peptidase-4 (DPP4), a serine exopeptidase . The nature of these interactions is largely dependent on the specific biochemical context and the other molecules involved.
Cellular Effects
The effects of Pyrrolidine-2-carboxamide on cells are diverse and can influence various cellular processes. For example, it has been suggested to have an impact on cell signaling pathways, gene expression, and cellular metabolism . The specific effects can vary depending on the cell type and the concentration of Pyrrolidine-2-carboxamide.
Molecular Mechanism
The molecular mechanism of action of Pyrrolidine-2-carboxamide involves its interactions with biomolecules at the molecular level. It can bind to certain enzymes, potentially leading to their inhibition or activation . Additionally, it may induce changes in gene expression .
Temporal Effects in Laboratory Settings
In laboratory settings, the effects of Pyrrolidine-2-carboxamide can change over time. This includes its stability, degradation, and any long-term effects on cellular function observed in in vitro or in vivo studies .
Dosage Effects in Animal Models
The effects of Pyrrolidine-2-carboxamide can vary with different dosages in animal models . This includes any threshold effects observed in these studies, as well as any toxic or adverse effects at high doses .
Metabolic Pathways
Pyrrolidine-2-carboxamide is involved in certain metabolic pathways, interacting with various enzymes or cofactors . This can also include any effects on metabolic flux or metabolite levels .
Transport and Distribution
Pyrrolidine-2-carboxamide is transported and distributed within cells and tissues . This includes any transporters or binding proteins it interacts with, as well as any effects on its localization or accumulation .
Subcellular Localization
This could include any targeting signals or post-translational modifications that direct it to specific compartments or organelles .
Vorbereitungsmethoden
Synthetic Routes and Reaction Conditions: Pyrrolidine-2-carboxamide can be synthesized through several methods. One common approach involves the reaction of pyrrolidine with chloroformamide under basic conditions. Another method includes the cyclization of N-substituted amino acids .
Industrial Production Methods: Industrial production often employs catalytic hydrogenation of pyrrolidine-2-carbonitrile in the presence of a suitable catalyst. This method ensures high yield and purity of the final product .
Analyse Chemischer Reaktionen
Types of Reactions: Pyrrolidine-2-carboxamide undergoes various chemical reactions, including:
Oxidation: It can be oxidized to form pyrrolidine-2,5-dione.
Reduction: Reduction reactions can yield pyrrolidine derivatives with different substituents.
Substitution: Nucleophilic substitution reactions can introduce various functional groups into the pyrrolidine ring.
Common Reagents and Conditions:
Oxidation: Common oxidizing agents include potassium permanganate and chromium trioxide.
Reduction: Catalytic hydrogenation using palladium on carbon.
Substitution: Reagents such as alkyl halides and acyl chlorides are frequently used.
Major Products:
Oxidation: Pyrrolidine-2,5-dione.
Reduction: Various substituted pyrrolidines.
Substitution: N-substituted pyrrolidine derivatives.
Vergleich Mit ähnlichen Verbindungen
- Pyrrolidine-2-one
- Pyrrolidine-2,5-dione
- Prolinol
Comparison: Pyrrolidine-2-carboxamide is unique due to its specific amide functional group, which imparts distinct chemical and biological properties. Compared to pyrrolidine-2-one and pyrrolidine-2,5-dione, pyrrolidine-2-carboxamide exhibits different reactivity and stability profiles. Prolinol, another similar compound, differs in its hydroxyl functional group, which affects its solubility and interaction with biological targets .
Eigenschaften
IUPAC Name |
pyrrolidine-2-carboxamide | |
---|---|---|
Source | PubChem | |
URL | https://pubchem.ncbi.nlm.nih.gov | |
Description | Data deposited in or computed by PubChem | |
InChI |
InChI=1S/C5H10N2O/c6-5(8)4-2-1-3-7-4/h4,7H,1-3H2,(H2,6,8) | |
Source | PubChem | |
URL | https://pubchem.ncbi.nlm.nih.gov | |
Description | Data deposited in or computed by PubChem | |
InChI Key |
VLJNHYLEOZPXFW-UHFFFAOYSA-N | |
Source | PubChem | |
URL | https://pubchem.ncbi.nlm.nih.gov | |
Description | Data deposited in or computed by PubChem | |
Canonical SMILES |
C1CC(NC1)C(=O)N | |
Source | PubChem | |
URL | https://pubchem.ncbi.nlm.nih.gov | |
Description | Data deposited in or computed by PubChem | |
Molecular Formula |
C5H10N2O | |
Source | PubChem | |
URL | https://pubchem.ncbi.nlm.nih.gov | |
Description | Data deposited in or computed by PubChem | |
DSSTOX Substance ID |
DTXSID20973828 | |
Record name | Pyrrolidine-2-carboximidic acid | |
Source | EPA DSSTox | |
URL | https://comptox.epa.gov/dashboard/DTXSID20973828 | |
Description | DSSTox provides a high quality public chemistry resource for supporting improved predictive toxicology. | |
Molecular Weight |
114.15 g/mol | |
Source | PubChem | |
URL | https://pubchem.ncbi.nlm.nih.gov | |
Description | Data deposited in or computed by PubChem | |
Solubility |
>17.1 [ug/mL] (The mean of the results at pH 7.4) | |
Record name | SID24834771 | |
Source | Burnham Center for Chemical Genomics | |
URL | https://pubchem.ncbi.nlm.nih.gov/bioassay/1996#section=Data-Table | |
Description | Aqueous solubility in buffer at pH 7.4 | |
CAS No. |
58274-20-7 | |
Record name | Pyrrolidine-2-carboximidic acid | |
Source | EPA DSSTox | |
URL | https://comptox.epa.gov/dashboard/DTXSID20973828 | |
Description | DSSTox provides a high quality public chemistry resource for supporting improved predictive toxicology. | |
Retrosynthesis Analysis
AI-Powered Synthesis Planning: Our tool employs the Template_relevance Pistachio, Template_relevance Bkms_metabolic, Template_relevance Pistachio_ringbreaker, Template_relevance Reaxys, Template_relevance Reaxys_biocatalysis model, leveraging a vast database of chemical reactions to predict feasible synthetic routes.
One-Step Synthesis Focus: Specifically designed for one-step synthesis, it provides concise and direct routes for your target compounds, streamlining the synthesis process.
Accurate Predictions: Utilizing the extensive PISTACHIO, BKMS_METABOLIC, PISTACHIO_RINGBREAKER, REAXYS, REAXYS_BIOCATALYSIS database, our tool offers high-accuracy predictions, reflecting the latest in chemical research and data.
Strategy Settings
Precursor scoring | Relevance Heuristic |
---|---|
Min. plausibility | 0.01 |
Model | Template_relevance |
Template Set | Pistachio/Bkms_metabolic/Pistachio_ringbreaker/Reaxys/Reaxys_biocatalysis |
Top-N result to add to graph | 6 |
Feasible Synthetic Routes
Q1: How does (2R,4S)-N-(2,5-difluorophenyl)-4-hydroxy-1-(2,2,2-trifluoroacetyl)pyrrolidine-2-carboxamide impact hepatocellular carcinoma cells?
A1: Research indicates that this specific pyrrolidine-2-carboxamide derivative inhibits the growth of HepG2 cells (a human hepatocellular carcinoma cell line) in a dose- and time-dependent manner. [, ] This effect is attributed to the compound's ability to induce apoptosis, a form of programmed cell death, in these cancer cells. [, ] Further investigation revealed that this compound modulates the expression of various genes involved in apoptosis, including those belonging to the TNF, BCL2, IAP, and caspase families. [] This suggests that the compound affects both the extrinsic and intrinsic apoptotic signaling pathways. []
Q2: What is the molecular formula and weight of 1-(4-methoxybenzenesulfonyl)-5-oxo-pyrrolidine-2-carboxamide?
A2: The molecular formula for this compound is C12H14O5N2S, and its molecular weight is 310.33 g/mol. []
Q3: How was the structure of (Sa,S)-N-[2′-(4-methylphenylsulfonamido)-1,1′-binaphthyl-2′-yl]pyrrolidine-2-carboxamide confirmed?
A3: While specific characterization methods weren't detailed in the provided research for this compound, similar compounds were confirmed using techniques like NMR spectroscopy and elemental analysis. [, , , , ]
Q4: Was the stability of vildagliptin, a pyrrolidine-2-carboxamide drug, investigated under various conditions?
A4: Yes, research extensively explored the stability of vildagliptin. It was found to degrade under acidic, basic, and oxidative conditions, particularly at elevated temperatures. [] Degradation kinetics followed pseudo-first-order kinetics. [] Furthermore, the study examined its stability in the presence of excipients like lactose, mannitol, magnesium stearate, and polyvinylpyrrolidone, revealing interactions mainly affecting the -NH- and CO groups of the drug molecule. []
Q5: Can pyrrolidine-2-carboxamide derivatives act as organocatalysts?
A5: Yes, certain derivatives, like (Sa,S)-N-[2′-(4-methylphenylsulfonamido)-1,1′-binaphthyl-2′-yl]pyrrolidine-2-carboxamide, demonstrate organocatalytic activity in direct asymmetric aldol reactions. []
Q6: Did researchers use computational methods to understand the binding of antagonists to the human V2-renal vasopressin receptor?
A6: Yes, molecular modeling was employed to visualize the binding site of six nonpeptide antagonists, including SR49059 [(2S)1-[(2R3S)-(5-chloro-3-(2 chlorophenyl)-1-(3,4-dimethoxybenzene-sulfonyl)-3-hydroxy-2,3-dihydro-1H-indole-2-carbonyl]-pyrrolidine-2-carboxamide)]. [] These models revealed that the binding site of these antagonists partially overlaps with that of arginine vasopressin, the natural ligand. [] Importantly, the models suggested that while arginine vasopressin binds to the extracellular surface of the V2 receptor, nonpeptide antagonists penetrate deeper into the receptor's transmembrane region. []
Q7: How does modifying the hydroxyl group in (S)-N-(2-(aminomethyl)-5-chlorobenzyl)-1-((R)-2-hydroxy-3,3-dimethylbutanoyl)pyrrolidine-2-carboxamide impact its properties?
A7: Replacing the hydroxyl group with heterocycles in this compound led to improved chemical stability and pharmacokinetic profiles without significantly compromising its in vitro potency as a thrombin inhibitor. [] This modification strategy resulted in the identification of compound 10, which showed good selectivity over related serine proteases and in vivo efficacy in a rat arteriovenous shunt model. []
Haftungsausschluss und Informationen zu In-Vitro-Forschungsprodukten
Bitte beachten Sie, dass alle Artikel und Produktinformationen, die auf BenchChem präsentiert werden, ausschließlich zu Informationszwecken bestimmt sind. Die auf BenchChem zum Kauf angebotenen Produkte sind speziell für In-vitro-Studien konzipiert, die außerhalb lebender Organismen durchgeführt werden. In-vitro-Studien, abgeleitet von dem lateinischen Begriff "in Glas", beinhalten Experimente, die in kontrollierten Laborumgebungen unter Verwendung von Zellen oder Geweben durchgeführt werden. Es ist wichtig zu beachten, dass diese Produkte nicht als Arzneimittel oder Medikamente eingestuft sind und keine Zulassung der FDA für die Vorbeugung, Behandlung oder Heilung von medizinischen Zuständen, Beschwerden oder Krankheiten erhalten haben. Wir müssen betonen, dass jede Form der körperlichen Einführung dieser Produkte in Menschen oder Tiere gesetzlich strikt untersagt ist. Es ist unerlässlich, sich an diese Richtlinien zu halten, um die Einhaltung rechtlicher und ethischer Standards in Forschung und Experiment zu gewährleisten.