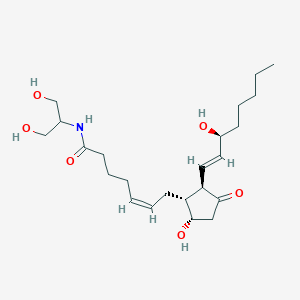
PGD2-Dihydroxypropanamin
Übersicht
Beschreibung
Prostaglandin D2 serinol amide is a bioactive lipid compound derived from prostaglandin D2. It is known for its role as a weak inhibitor of the hydrolysis of [3H]2-oleoylglycerol . This compound exhibits cannabinoid agonist activity at the cannabinoid receptor 1 and is considered an important endogenous monoglyceride species . Prostaglandin D2 serinol amide is involved in various physiological processes, including inflammation, pain modulation, and immune response.
Wissenschaftliche Forschungsanwendungen
Chemistry and Biochemistry
PGD2-SA is utilized as a model compound to study the synthesis and reactivity of bioactive lipids. Its chemical structure allows researchers to explore lipid metabolism and the interactions between various biomolecules. The compound acts as a weak inhibitor of the hydrolysis of [3H]2-oleoylglycerol, which provides insights into lipid signaling pathways and their physiological implications.
Biology
In biological research, PGD2-SA is investigated for its role in modulating inflammation, pain, and immune responses. It primarily targets the Prostaglandin D2 receptor 2 (DP2), leading to significant inflammatory cell chemotaxis and degranulation. This receptor interaction is crucial for understanding how PGD2-SA can influence conditions characterized by type 2 inflammation, such as asthma and allergic reactions .
Medicine
PGD2-SA shows potential therapeutic applications in treating inflammatory diseases, asthma, and pain management. Its ability to modulate immune responses makes it a candidate for developing new treatments aimed at conditions where prostaglandin signaling is disrupted or altered. Ongoing studies are exploring its efficacy in clinical settings .
Industrial Applications
In the pharmaceutical industry, PGD2-SA is being researched for its applications in drug development targeting specific prostaglandin receptors. Its stability and biological activity make it an attractive candidate for formulating new therapeutics that can effectively manage inflammatory conditions .
Target Interaction
PGD2-SA interacts predominantly with the DP2 receptor, initiating a cascade of biochemical reactions that result in increased production of PGD2. This interaction enhances inflammatory cell chemotaxis and degranulation, contributing to its biological effects.
Biochemical Pathways
The activation of the DP2 receptor by PGD2-SA leads to various downstream effects, including modulation of gene expression related to inflammation and pain perception. The compound's role in these pathways underscores its importance in both basic research and therapeutic development.
Inflammation Modulation
Research has demonstrated that PGD2-SA can effectively modulate inflammatory responses in animal models. In one study, varying dosages of PGD2-SA were administered to assess its impact on stress-induced analgesia, revealing dosage-dependent effects on pain perception.
Asthma Treatment Potential
A clinical study investigated the effects of PGD2-SA on asthma patients. Results indicated that the compound could reduce airway hyperresponsiveness by modulating immune cell activity through DP2 receptor engagement, suggesting its potential as a therapeutic agent.
Wirkmechanismus
Target of Action
PGD2-dihydroxypropanylamine, also known as Prostaglandin D2 serinol amide, primarily targets the Prostaglandin D2 receptor 2 (DP2) . This receptor is predominantly expressed by key cells involved in type 2 immune responses, including T H 2 cells, type 2 innate lymphoid cells, and eosinophils .
Mode of Action
The compound interacts with its target, the DP2 receptor, inducing significant inflammatory cell chemotaxis and degranulation . This interaction triggers the release of pro-inflammatory cytokines and harmful cationic proteases .
Biochemical Pathways
The activation of the DP2 receptor pathway leads to a cascade of biochemical reactions. Increased PGD2 production, facilitated by the interaction with the DP2 receptor, results in significant inflammatory cell chemotaxis and degranulation . This has serious consequences in the pulmonary milieu, leading to the release of pro-inflammatory cytokines and harmful cationic proteases .
Result of Action
The result of PGD2-dihydroxypropanylamine’s action is a significant increase in inflammatory cell chemotaxis and degranulation . This leads to tissue remodeling, mucus production, structural damage, and compromised lung function . These effects are particularly relevant in the context of diseases characterized by chronic inflammation, such as asthma .
Biochemische Analyse
Biochemical Properties
Prostaglandin D2 serinol amide plays a crucial role in biochemical reactions by interacting with various enzymes, proteins, and other biomolecules. It is known to be a weak inhibitor of the hydrolysis of [3H]2-oleoylglycerol . This interaction suggests that Prostaglandin D2 serinol amide can modulate the activity of enzymes involved in lipid metabolism. Additionally, it interacts with prostaglandin receptors, particularly the DP1 and DP2 receptors, which are involved in various physiological responses, including inflammation and immune regulation .
Cellular Effects
Prostaglandin D2 serinol amide exerts significant effects on various cell types and cellular processes. It influences cell function by modulating cell signaling pathways, gene expression, and cellular metabolism. For instance, it has been shown to play a role in the regulation of type 2 inflammation, which involves various immune cells such as T helper type 2 cells, eosinophils, and mast cells . By interacting with these cells, Prostaglandin D2 serinol amide can influence the production of cytokines and other inflammatory mediators, thereby affecting immune responses and inflammation .
Molecular Mechanism
The molecular mechanism of action of Prostaglandin D2 serinol amide involves its binding interactions with specific biomolecules and its ability to modulate enzyme activity. It acts as a weak inhibitor of the hydrolysis of [3H]2-oleoylglycerol, suggesting that it can interfere with lipid metabolism . Additionally, Prostaglandin D2 serinol amide interacts with prostaglandin receptors, particularly the DP1 and DP2 receptors, which are involved in mediating its effects on inflammation and immune responses . These interactions can lead to changes in gene expression and the activation or inhibition of specific signaling pathways.
Temporal Effects in Laboratory Settings
In laboratory settings, the effects of Prostaglandin D2 serinol amide can change over time due to its stability and degradation. Studies have shown that it can influence stress-induced analgesia in animal models, with its effects varying depending on the duration of exposure . For instance, acute administration of Prostaglandin D2 serinol amide can reverse stress-induced analgesia in male rats, while chronic exposure may lead to different outcomes . These temporal effects highlight the importance of considering the duration of exposure when studying the compound’s effects.
Dosage Effects in Animal Models
The effects of Prostaglandin D2 serinol amide vary with different dosages in animal models. Studies have demonstrated that different dosages can lead to varying outcomes, including threshold effects and potential toxic or adverse effects at high doses . For example, intrathecal administration of Prostaglandin D2 serinol amide at specific dosages can modulate stress-induced analgesia in male rats, with higher doses potentially leading to adverse effects . These findings underscore the need for careful dosage considerations in experimental settings.
Metabolic Pathways
Prostaglandin D2 serinol amide is involved in several metabolic pathways, including those related to lipid metabolism. It is a stable analog of prostaglandin D2 2-glyceryl ester and can be metabolized by cyclooxygenase-2 (COX-2) and specific prostaglandin synthases . These metabolic pathways are crucial for the compound’s biological activity and its role in modulating inflammatory responses and other physiological processes .
Transport and Distribution
The transport and distribution of Prostaglandin D2 serinol amide within cells and tissues involve specific transporters and binding proteins. It is known to interact with prostaglandin receptors, which facilitate its distribution and localization within target tissues . Additionally, the compound’s stability and solubility properties influence its transport and accumulation in different cellular compartments .
Subcellular Localization
Prostaglandin D2 serinol amide’s subcellular localization is influenced by its interactions with specific biomolecules and its post-translational modifications. It is known to localize within specific cellular compartments, where it can exert its effects on enzyme activity and signaling pathways . The compound’s targeting signals and modifications play a crucial role in directing it to specific organelles, thereby influencing its biological activity and function.
Vorbereitungsmethoden
Synthetische Wege und Reaktionsbedingungen: Die Synthese von Prostaglandin D2-Serinol-Amid beinhaltet typischerweise die Cyclooxygenierung der Arachidonoyl-Seitenkette von 1-Arachidonoylglycerol und verwandten Verbindungen . Dieser Prozess blockiert ihre Fähigkeit, den Metabolismus von Anandamid und 2-Oleoylglycerol durch Rattenhirn in vitro zu verhindern . Der synthetische Weg umfasst die Verwendung spezifischer Prostaglandin-Synthasen und Cyclooxygenase-2-Enzyme zur Bildung von Prostaglandin D2-Glycerylestern .
Industrielle Produktionsmethoden: Die industrielle Produktion von Prostaglandin D2-Serinol-Amid beinhaltet die Verwendung fortschrittlicher biotechnologischer Methoden, um eine hohe Reinheit und Ausbeute zu gewährleisten. Der Prozess umfasst die Extraktion und Reinigung der Verbindung aus biologischen Quellen, gefolgt von chemischer Synthese und Verfeinerung, um die gewünschten Produktspezifikationen zu erreichen .
Analyse Chemischer Reaktionen
Arten von Reaktionen: Prostaglandin D2-Serinol-Amid unterliegt verschiedenen chemischen Reaktionen, darunter Oxidation, Reduktion und Substitution. Diese Reaktionen sind essentiell für seine biologische Aktivität und therapeutische Anwendungen.
Häufige Reagenzien und Bedingungen:
Oxidation: Die Verbindung kann unter kontrollierten Bedingungen mit Reagenzien wie Kaliumpermanganat oder Wasserstoffperoxid oxidiert werden.
Reduktion: Reduktionsreaktionen können mit Reduktionsmitteln wie Natriumborhydrid oder Lithiumaluminiumhydrid durchgeführt werden.
Substitution: Substitutionsreaktionen beinhalten den Austausch von funktionellen Gruppen mit Reagenzien wie Halogenen oder Nukleophilen.
Hauptprodukte, die gebildet werden: Die Hauptprodukte, die aus diesen Reaktionen gebildet werden, sind Prostaglandin D2-Glycerylester und andere verwandte Verbindungen, die ähnliche biologische Aktivitäten aufweisen .
Vergleich Mit ähnlichen Verbindungen
- Prostaglandin D2
- Prostaglandin E2
- Prostaglandin F2α
- Prostaglandin I2
Comparison: Prostaglandin D2 serinol amide is unique due to its specific receptor interactions and its role as a weak inhibitor of the hydrolysis of [3H]2-oleoylglycerol . Unlike other prostaglandins, it exhibits cannabinoid agonist activity at the cannabinoid receptor 1, making it a valuable compound for studying the endocannabinoid system . Additionally, its stability as a prostaglandin D2-glyceryl ester analog sets it apart from other prostaglandin derivatives .
Biologische Aktivität
Prostaglandin D2 serinol amide (PGD2-SA) is a derivative of prostaglandin D2 (PGD2), which is known for its significant biological activities, particularly in inflammatory responses and sleep regulation. This article explores the biological activity of PGD2-SA, including its synthesis, mechanisms of action, and potential therapeutic applications.
Overview of Prostaglandin D2
Prostaglandin D2 is primarily produced by mast cells and plays a crucial role in various physiological processes, including:
- Vasodilation : PGD2 induces vasodilation and increases vascular permeability.
- Bronchoconstriction : It contributes to airway constriction during allergic reactions.
- Sleep Promotion : PGD2 is recognized as a potent endogenous sleep-promoting substance .
Synthesis of Prostaglandin D2 Serinol Amide
PGD2-SA is synthesized through the modification of PGD2, incorporating a serinol amide moiety. This structural alteration aims to enhance the stability and efficacy of the compound compared to its parent molecule. The synthesis typically involves:
- Starting Material : Prostaglandin D2.
- Reagents : Serinol and coupling agents are used to facilitate the formation of the amide bond.
- Purification : The final product is purified using chromatography techniques.
Inflammatory Response Modulation
PGD2-SA has been shown to exhibit anti-inflammatory properties similar to those of PGD2. Research indicates that it can modulate various immune responses, particularly in conditions such as asthma and allergic rhinitis. In animal models, PGD2 receptor antagonists have demonstrated efficacy in reducing eosinophil infiltration and suppressing allergic responses .
Sleep Regulation
As a derivative of PGD2, PGD2-SA may retain the sleep-promoting effects associated with its parent compound. Studies have demonstrated that PGD2 influences sleep-wake cycles through its action on specific receptors (DP1 and DP2) in the brain . The potential for PGD2-SA to enhance these effects warrants further investigation.
Efficacy in Allergic Models
In vivo studies involving PGD2 receptor antagonists have provided insight into the biological activity of related compounds. For example:
- Model : Allergic rhinitis in mice.
- Findings : Compounds similar to PGD2-SA effectively reduced symptoms by inhibiting eosinophil migration and cytokine production (IL-4, IL-5) associated with Th2 responses .
Study | Model | Results |
---|---|---|
Allergic Rhinitis | Significant reduction in eosinophil infiltration | |
Asthma | Suppression of inflammatory cytokines |
Potential Therapeutic Applications
Given its biological activities, PGD2-SA may have therapeutic potential in treating conditions characterized by excessive inflammation or sleep disorders. Future research should focus on:
- Pharmacokinetics : Understanding absorption, distribution, metabolism, and excretion.
- Clinical Trials : Evaluating safety and efficacy in human subjects.
Eigenschaften
IUPAC Name |
(Z)-N-(1,3-dihydroxypropan-2-yl)-7-[(1R,2R,5S)-5-hydroxy-2-[(E,3S)-3-hydroxyoct-1-enyl]-3-oxocyclopentyl]hept-5-enamide | |
---|---|---|
Source | PubChem | |
URL | https://pubchem.ncbi.nlm.nih.gov | |
Description | Data deposited in or computed by PubChem | |
InChI |
InChI=1S/C23H39NO6/c1-2-3-6-9-18(27)12-13-20-19(21(28)14-22(20)29)10-7-4-5-8-11-23(30)24-17(15-25)16-26/h4,7,12-13,17-21,25-28H,2-3,5-6,8-11,14-16H2,1H3,(H,24,30)/b7-4-,13-12+/t18-,19+,20+,21-/m0/s1 | |
Source | PubChem | |
URL | https://pubchem.ncbi.nlm.nih.gov | |
Description | Data deposited in or computed by PubChem | |
InChI Key |
YRUGYYZQISUWGN-AVMYJHFGSA-N | |
Source | PubChem | |
URL | https://pubchem.ncbi.nlm.nih.gov | |
Description | Data deposited in or computed by PubChem | |
Canonical SMILES |
CCCCCC(C=CC1C(C(CC1=O)O)CC=CCCCC(=O)NC(CO)CO)O | |
Source | PubChem | |
URL | https://pubchem.ncbi.nlm.nih.gov | |
Description | Data deposited in or computed by PubChem | |
Isomeric SMILES |
CCCCC[C@@H](/C=C/[C@@H]1[C@H]([C@H](CC1=O)O)C/C=C\CCCC(=O)NC(CO)CO)O | |
Source | PubChem | |
URL | https://pubchem.ncbi.nlm.nih.gov | |
Description | Data deposited in or computed by PubChem | |
Molecular Formula |
C23H39NO6 | |
Source | PubChem | |
URL | https://pubchem.ncbi.nlm.nih.gov | |
Description | Data deposited in or computed by PubChem | |
Molecular Weight |
425.6 g/mol | |
Source | PubChem | |
URL | https://pubchem.ncbi.nlm.nih.gov | |
Description | Data deposited in or computed by PubChem | |
Retrosynthesis Analysis
AI-Powered Synthesis Planning: Our tool employs the Template_relevance Pistachio, Template_relevance Bkms_metabolic, Template_relevance Pistachio_ringbreaker, Template_relevance Reaxys, Template_relevance Reaxys_biocatalysis model, leveraging a vast database of chemical reactions to predict feasible synthetic routes.
One-Step Synthesis Focus: Specifically designed for one-step synthesis, it provides concise and direct routes for your target compounds, streamlining the synthesis process.
Accurate Predictions: Utilizing the extensive PISTACHIO, BKMS_METABOLIC, PISTACHIO_RINGBREAKER, REAXYS, REAXYS_BIOCATALYSIS database, our tool offers high-accuracy predictions, reflecting the latest in chemical research and data.
Strategy Settings
Precursor scoring | Relevance Heuristic |
---|---|
Min. plausibility | 0.01 |
Model | Template_relevance |
Template Set | Pistachio/Bkms_metabolic/Pistachio_ringbreaker/Reaxys/Reaxys_biocatalysis |
Top-N result to add to graph | 6 |
Feasible Synthetic Routes
Haftungsausschluss und Informationen zu In-Vitro-Forschungsprodukten
Bitte beachten Sie, dass alle Artikel und Produktinformationen, die auf BenchChem präsentiert werden, ausschließlich zu Informationszwecken bestimmt sind. Die auf BenchChem zum Kauf angebotenen Produkte sind speziell für In-vitro-Studien konzipiert, die außerhalb lebender Organismen durchgeführt werden. In-vitro-Studien, abgeleitet von dem lateinischen Begriff "in Glas", beinhalten Experimente, die in kontrollierten Laborumgebungen unter Verwendung von Zellen oder Geweben durchgeführt werden. Es ist wichtig zu beachten, dass diese Produkte nicht als Arzneimittel oder Medikamente eingestuft sind und keine Zulassung der FDA für die Vorbeugung, Behandlung oder Heilung von medizinischen Zuständen, Beschwerden oder Krankheiten erhalten haben. Wir müssen betonen, dass jede Form der körperlichen Einführung dieser Produkte in Menschen oder Tiere gesetzlich strikt untersagt ist. Es ist unerlässlich, sich an diese Richtlinien zu halten, um die Einhaltung rechtlicher und ethischer Standards in Forschung und Experiment zu gewährleisten.