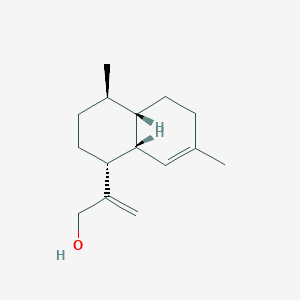
Artemisinic alcohol
Übersicht
Beschreibung
Artemisinic alcohol is a sesquiterpene alcohol derived from the plant Artemisia annua, commonly known as sweet wormwood. It is an intermediate in the biosynthesis of artemisinin, a potent antimalarial compound. This compound plays a crucial role in the production of artemisinin and its derivatives, which are widely used in the treatment of malaria and other diseases.
Wissenschaftliche Forschungsanwendungen
Artemisinic alcohol has several scientific research applications across various fields:
Chemistry:
- Used as an intermediate in the synthesis of artemisinin and its derivatives.
- Studied for its potential to undergo various chemical transformations to yield novel compounds with potential therapeutic applications.
Biology:
- Investigated for its role in the biosynthesis of artemisinin in Artemisia annua.
- Used in studies to understand the metabolic pathways involved in the production of sesquiterpene lactones.
Medicine:
- Plays a crucial role in the production of artemisinin-based combination therapies (ACTs) for the treatment of malaria.
- Explored for its potential anticancer, antiviral, and anti-inflammatory properties .
Industry:
Wirkmechanismus
Target of Action
Artemisinic alcohol, also known as (+)-artemisinic alcohol, is a bioactive molecule derived from the extracts of Artemisia annua L. . It is primarily targeted against the malaria parasite Plasmodium falciparum . The compound’s efficacy extends beyond malaria to a variety of human diseases such as cancer, tuberculosis, viral diseases, immune diseases, and parasitic infections like schistosomiasis .
Mode of Action
The mode of action of this compound involves a series of interactions with its targets. The compound is believed to interact with heat shock protein (HSP70) and a hypoxanthine phosphoribosyltransferase (PF10_0121) related to purine biosynthesis . These interactions result in decreased sensitivity to artemisinin .
Biochemical Pathways
The biosynthesis of this compound involves two independent pathways producing isopentenyl pyrophosphate (IPP): the cytosolic mevalonate pathway (MVA) and the plastidial methylerythritol phosphate (MEP) pathway . In the MVA pathway, HMG-CoA reductase (HMGR) is the rate-limiting enzyme, while in the MEP pathway, DXR is the rate-limiting enzyme . The compound amorpha-4,11-diene is converted into this compound by the action of amorphadiene monooxygenase, an enzyme of cytochrome P450 .
Pharmacokinetics
It is known that artemisinin, a sesquiterpene lactone, contains 15 carbon atoms with lipophilic properties , which may influence the bioavailability of this compound.
Result of Action
The molecular and cellular effects of this compound’s action are primarily its potent anti-malarial effects . It has also shown significant effects against different diseases such as cancer, tuberculosis, autoimmune diseases, schistosomiasis, and viral diseases .
Action Environment
The action, efficacy, and stability of this compound can be influenced by various environmental factors. For instance, the production of this compound in Artemisia annua can be affected by the plant’s growth conditions . Furthermore, the expression of genes involved in the biosynthesis of this compound can be influenced by diverse plant hormone or environmental signaling routes .
Biochemische Analyse
Biochemical Properties
Artemisinic alcohol is involved in several biochemical reactions within the artemisinin biosynthetic pathway. It is produced from amorpha-4,11-diene through the action of cytochrome P450 monooxygenase (CYP71AV1) and its redox partner, cytochrome P450 reductase (CPR). The compound is then further oxidized to artemisinic aldehyde and artemisinic acid by the same enzymes . These interactions highlight the importance of cytochrome P450 enzymes in the conversion of this compound to its subsequent products.
Cellular Effects
This compound influences various cellular processes, particularly in the context of its role in the biosynthesis of artemisinin. It has been shown to affect the expression of genes involved in the artemisinin pathway, thereby impacting the overall production of artemisinin in cells . Additionally, the compound’s interaction with cytochrome P450 enzymes suggests its involvement in cellular detoxification processes and the regulation of cellular metabolism.
Molecular Mechanism
At the molecular level, this compound exerts its effects through binding interactions with cytochrome P450 enzymes. The compound is hydroxylated by CYP71AV1, leading to the formation of artemisinic aldehyde and artemisinic acid . This enzymatic activity is crucial for the progression of the artemisinin biosynthetic pathway. Furthermore, the regulation of gene expression by this compound involves the activation of transcription factors that control the expression of enzymes in the pathway .
Temporal Effects in Laboratory Settings
In laboratory settings, the stability and degradation of this compound have been studied to understand its temporal effects. The compound is relatively stable under controlled conditions, but its conversion to artemisinic aldehyde and artemisinic acid can be influenced by factors such as enzyme activity and environmental conditions . Long-term studies have shown that the presence of this compound can lead to sustained production of artemisinin in engineered plant systems .
Dosage Effects in Animal Models
The effects of this compound at different dosages have been investigated in animal models to determine its therapeutic potential and toxicity. Studies have shown that low to moderate doses of this compound can enhance the production of artemisinin without causing significant adverse effects . High doses of the compound may lead to toxicity and negative impacts on cellular function, highlighting the importance of dosage optimization in therapeutic applications.
Metabolic Pathways
This compound is a key intermediate in the artemisinin biosynthetic pathway. It is produced from amorpha-4,11-diene and subsequently converted to artemisinic aldehyde and artemisinic acid by cytochrome P450 enzymes . The compound’s involvement in this pathway underscores its importance in the overall production of artemisinin and its derivatives. Additionally, this compound interacts with various cofactors and enzymes that regulate metabolic flux and metabolite levels in the pathway .
Transport and Distribution
Within cells, this compound is transported and distributed through interactions with specific transporters and binding proteins. These interactions facilitate the movement of the compound to different cellular compartments where it undergoes further enzymatic conversion . The distribution of this compound within tissues is also influenced by its binding affinity to cellular proteins and its solubility in cellular membranes .
Subcellular Localization
This compound is localized in specific subcellular compartments, including the endoplasmic reticulum and peroxisomes, where it interacts with cytochrome P450 enzymes . The targeting of this compound to these compartments is mediated by targeting signals and post-translational modifications that direct the compound to its site of action. This subcellular localization is essential for the efficient conversion of this compound to its subsequent products in the artemisinin biosynthetic pathway .
Vorbereitungsmethoden
Synthetic Routes and Reaction Conditions: Artemisinic alcohol can be synthesized through various methods, including chemical synthesis and biotechnological approaches. One common method involves the oxidation of amorpha-4,11-diene to this compound using cytochrome P450 monooxygenase (CYP71AV1) and cytochrome P450 reductase (CPR) under controlled conditions . The reaction typically occurs in the presence of oxygen and requires specific cofactors for optimal enzyme activity.
Industrial Production Methods: Industrial production of this compound often involves the use of genetically engineered microorganisms, such as Saccharomyces cerevisiae (yeast). These microorganisms are engineered to express the necessary enzymes for the biosynthesis of this compound from simple carbon sources. The fermentation process is optimized to achieve high yields of this compound, which can then be further converted to artemisinin .
Analyse Chemischer Reaktionen
Types of Reactions: Artemisinic alcohol undergoes several types of chemical reactions, including oxidation, reduction, and substitution.
Common Reagents and Conditions:
Substitution: Substitution reactions involving this compound can lead to the formation of various derivatives with potential biological activities.
Major Products:
Artemisinic aldehyde: Formed through the oxidation of this compound.
Artemisinic acid: Further oxidation of artemisinic aldehyde.
Dihydrothis compound: Formed through the reduction of this compound.
Vergleich Mit ähnlichen Verbindungen
Dihydroartemisinic alcohol: An intermediate in the biosynthesis of artemisinin.
Artemisinic aldehyde: An oxidation product of this compound.
Artemisinic acid: A further oxidation product of artemisinic aldehyde.
This compound’s uniqueness lies in its specific role in the biosynthesis pathway of artemisinin, making it a critical compound for the production of this life-saving drug.
Eigenschaften
IUPAC Name |
2-[(1R,4R,4aS,8aR)-4,7-dimethyl-1,2,3,4,4a,5,6,8a-octahydronaphthalen-1-yl]prop-2-en-1-ol | |
---|---|---|
Source | PubChem | |
URL | https://pubchem.ncbi.nlm.nih.gov | |
Description | Data deposited in or computed by PubChem | |
InChI |
InChI=1S/C15H24O/c1-10-4-6-13-11(2)5-7-14(12(3)9-16)15(13)8-10/h8,11,13-16H,3-7,9H2,1-2H3/t11-,13+,14+,15+/m1/s1 | |
Source | PubChem | |
URL | https://pubchem.ncbi.nlm.nih.gov | |
Description | Data deposited in or computed by PubChem | |
InChI Key |
CZSSHKCZSDDOAH-UNQGMJICSA-N | |
Source | PubChem | |
URL | https://pubchem.ncbi.nlm.nih.gov | |
Description | Data deposited in or computed by PubChem | |
Canonical SMILES |
CC1CCC(C2C1CCC(=C2)C)C(=C)CO | |
Source | PubChem | |
URL | https://pubchem.ncbi.nlm.nih.gov | |
Description | Data deposited in or computed by PubChem | |
Isomeric SMILES |
C[C@@H]1CC[C@H]([C@@H]2[C@H]1CCC(=C2)C)C(=C)CO | |
Source | PubChem | |
URL | https://pubchem.ncbi.nlm.nih.gov | |
Description | Data deposited in or computed by PubChem | |
Molecular Formula |
C15H24O | |
Source | PubChem | |
URL | https://pubchem.ncbi.nlm.nih.gov | |
Description | Data deposited in or computed by PubChem | |
DSSTOX Substance ID |
DTXSID201315937 | |
Record name | Arteannuic alcohol | |
Source | EPA DSSTox | |
URL | https://comptox.epa.gov/dashboard/DTXSID201315937 | |
Description | DSSTox provides a high quality public chemistry resource for supporting improved predictive toxicology. | |
Molecular Weight |
220.35 g/mol | |
Source | PubChem | |
URL | https://pubchem.ncbi.nlm.nih.gov | |
Description | Data deposited in or computed by PubChem | |
CAS No. |
125184-95-4 | |
Record name | Arteannuic alcohol | |
Source | CAS Common Chemistry | |
URL | https://commonchemistry.cas.org/detail?cas_rn=125184-95-4 | |
Description | CAS Common Chemistry is an open community resource for accessing chemical information. Nearly 500,000 chemical substances from CAS REGISTRY cover areas of community interest, including common and frequently regulated chemicals, and those relevant to high school and undergraduate chemistry classes. This chemical information, curated by our expert scientists, is provided in alignment with our mission as a division of the American Chemical Society. | |
Explanation | The data from CAS Common Chemistry is provided under a CC-BY-NC 4.0 license, unless otherwise stated. | |
Record name | Arteannuic alcohol | |
Source | EPA DSSTox | |
URL | https://comptox.epa.gov/dashboard/DTXSID201315937 | |
Description | DSSTox provides a high quality public chemistry resource for supporting improved predictive toxicology. | |
Retrosynthesis Analysis
AI-Powered Synthesis Planning: Our tool employs the Template_relevance Pistachio, Template_relevance Bkms_metabolic, Template_relevance Pistachio_ringbreaker, Template_relevance Reaxys, Template_relevance Reaxys_biocatalysis model, leveraging a vast database of chemical reactions to predict feasible synthetic routes.
One-Step Synthesis Focus: Specifically designed for one-step synthesis, it provides concise and direct routes for your target compounds, streamlining the synthesis process.
Accurate Predictions: Utilizing the extensive PISTACHIO, BKMS_METABOLIC, PISTACHIO_RINGBREAKER, REAXYS, REAXYS_BIOCATALYSIS database, our tool offers high-accuracy predictions, reflecting the latest in chemical research and data.
Strategy Settings
Precursor scoring | Relevance Heuristic |
---|---|
Min. plausibility | 0.01 |
Model | Template_relevance |
Template Set | Pistachio/Bkms_metabolic/Pistachio_ringbreaker/Reaxys/Reaxys_biocatalysis |
Top-N result to add to graph | 6 |
Feasible Synthetic Routes
Q1: What is the role of artemisinic alcohol in the biosynthesis of artemisinin?
A: this compound is formed from the cyclization of farnesyl pyrophosphate by amorpha-4,11-diene synthase (ADS), followed by oxidation by the cytochrome P450 enzyme, amorpha-4,11-diene 12-monooxygenase (CYP71AV1) [, , ]. It serves as a precursor to artemisinic aldehyde, which is subsequently reduced to dihydroartemisinic aldehyde and then oxidized to dihydroartemisinic acid. Dihydroartemisinic acid can then be converted non-enzymatically into artemisinin [, ].
Q2: Can this compound be produced in organisms other than Artemisia annua?
A: Yes, research has shown that this compound can be produced in genetically modified organisms. For example, heterologous expression of A. annua ADS and CYP71AV1 in tobacco plants led to the accumulation of amorphadiene and this compound []. Similarly, metabolic engineering of Saccharomyces cerevisiae with genes encoding for ADS, CYP71AV1, this compound dehydrogenase (ADH1), artemisinic aldehyde Δ11(13) reductase (DBR2), and aldehyde dehydrogenase 1 (ALDH1) successfully produced this compound and dihydroartemisinic acid [].
Q3: What is the significance of producing this compound in other organisms?
A: Producing artemisinin precursors like this compound in alternative systems, such as tobacco or yeast, could potentially provide a more cost-effective and sustainable source of artemisinin than relying solely on Artemisia annua cultivation [, ].
Q4: Are there any challenges in producing this compound through heterologous expression?
A: Yes, challenges exist in optimizing the metabolic pathways in engineered organisms. For example, in tobacco, the accumulation of this compound was observed, but not artemisinic acid, suggesting that the conditions within the transgenic tobacco cells favored reduction to alcohols rather than oxidation to acids []. Similar issues with balancing metabolic flux were observed in yeast [].
Q5: Beyond genetic modification, are there other ways to produce artemisinic acid?
A: Yes, chemical synthesis provides an alternative approach. Researchers have successfully utilized potassium tetramethylpiperidide (KTMP) for the site-selective deprotonation of amorphadiene, leading to the formation of this compound. This method allows for the large-scale chemical synthesis of artemisinic acid from readily available starting materials [].
Q6: What is the significance of studying artemisinin biosynthesis in non-artemisinin-producing Artemisia species?
A: Investigating the presence and function of artemisinin biosynthetic enzyme homologs in other Artemisia species offers insights into the evolution of this pathway and may reveal novel enzymatic activities with potential applications in synthetic biology and metabolic engineering [].
Q7: How does the distribution of artemisinin and its precursors differ between living and dead Artemisia annua leaves?
A: Research indicates that while the total concentration of artemisinin and its precursors is initially higher in green leaves, the conversion of precursors to artemisinin is more efficient in dead leaves. Consequently, mature dead leaves tend to have a higher concentration of artemisinin than green leaves, highlighting their importance for overall yield [].
Haftungsausschluss und Informationen zu In-Vitro-Forschungsprodukten
Bitte beachten Sie, dass alle Artikel und Produktinformationen, die auf BenchChem präsentiert werden, ausschließlich zu Informationszwecken bestimmt sind. Die auf BenchChem zum Kauf angebotenen Produkte sind speziell für In-vitro-Studien konzipiert, die außerhalb lebender Organismen durchgeführt werden. In-vitro-Studien, abgeleitet von dem lateinischen Begriff "in Glas", beinhalten Experimente, die in kontrollierten Laborumgebungen unter Verwendung von Zellen oder Geweben durchgeführt werden. Es ist wichtig zu beachten, dass diese Produkte nicht als Arzneimittel oder Medikamente eingestuft sind und keine Zulassung der FDA für die Vorbeugung, Behandlung oder Heilung von medizinischen Zuständen, Beschwerden oder Krankheiten erhalten haben. Wir müssen betonen, dass jede Form der körperlichen Einführung dieser Produkte in Menschen oder Tiere gesetzlich strikt untersagt ist. Es ist unerlässlich, sich an diese Richtlinien zu halten, um die Einhaltung rechtlicher und ethischer Standards in Forschung und Experiment zu gewährleisten.