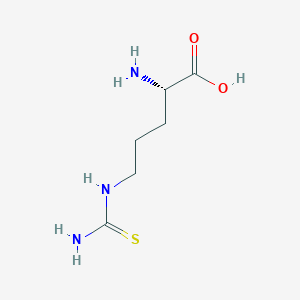
L-thiocitrulline
Übersicht
Beschreibung
L-thiocitrulline is a potent inhibitor of nitric-oxide synthases (NOS), which are enzymes responsible for the oxidation of L-arginine to citrulline and nitric oxide (NO). It is a stereospecific compound that has been shown to bind to the heme cofactor region of NOS, affecting the enzyme's activity. L-thiocitrulline has been studied for its potential therapeutic applications, particularly in conditions associated with the overproduction of NO, such as septic shock [
Wissenschaftliche Forschungsanwendungen
Role in Cellular Redox Homeostasis
L-thiocitrulline is involved in the maintenance of cellular redox homeostasis. It plays a crucial role in plant responses to stress factors and regulates cellular metabolism. This compound is part of the class of low-molecular-weight (LMW) thiols, which are highly reactive and essential for maintaining the redox balance within cells. LMW thiols, including L-thiocitrulline, are involved in post-translational protein modification and are pivotal in the plant's response to various stress factors (Pivato, Fabrega-Prats, & Masi, 2014).
Understanding Pharmacokinetics and Drug Safety
Understanding the pharmacokinetics and safety profile of drugs is crucial for effective therapy. L-thiocitrulline and related compounds are examined to gain insights into drug action mechanisms and address unexplained cases of drug toxicity or treatment failure. Such studies aim to enhance the efficacy and minimize the toxicity of treatments by tailoring therapy based on the expression profile of factors involved in drug activation pathways. This approach is especially relevant in the context of thiopurines, a class of drugs that includes L-thiocitrulline-related compounds (Fotoohi, Coulthard, & Albertioni, 2010).
In-depth Study of Drug Safety and Toxicity
A comprehensive understanding of drug safety and toxicity is essential for the appropriate use of any pharmacological agent. In the context of thiopurines (a class related to L-thiocitrulline), a detailed review of their safety and toxicity profiles is crucial. This involves discussing adverse drug events, emphasizing the significance of drug interactions, and considering the relative safety of thiopurine use across different patient categories such as adults, the elderly, children, and pregnant women. The goal is to ensure that drug safety, clinical response, and the balance of risks and benefits are considered throughout the treatment duration for different patient groups. Regular clinical follow-ups and monitoring are part of this comprehensive approach to drug safety (Konidari & El Matary, 2014).
Contribution to Environmental Impact Assessments
L-thiocitrulline and related compounds play a role in environmental impact assessments, particularly in the context of Low-Impact Development (LID). The Long-Term Hydrologic Impact Assessment (L-THIA) model, for instance, uses data about land use, soil type, and climatic factors to estimate runoff and pollutant loadings. This model is crucial for watershed-scale analysis and helps in understanding the environmental impacts of land use changes and the effectiveness of various designs and LID practices. While not directly mentioning L-thiocitrulline, studies related to the L-THIA model highlight the importance of considering the environmental impact of chemicals and development practices (Cai, Zhu, Ruggiero, Newman, & Horney, 2023).
Wirkmechanismus
Target of Action
L-Thiocitrulline primarily targets the enzyme Nitric Oxide Synthase (NOS), specifically the inducible isoform . NOS is responsible for the production of nitric oxide (NO), a messenger molecule with diverse functions throughout the body . In macrophages, NO mediates tumoricidal and bactericidal actions .
Mode of Action
L-Thiocitrulline acts as a potent, stereospecific inhibitor of NOS . It competes with L-arginine, the natural substrate of NOS, indicating that its initial binding is as a substrate/product analog . Unlike L-arginine and Nω-methyl-L-arginine, another NOS inhibitor, L-Thiocitrulline binding elicits a ‘Type II’ difference spectrum, indicating a high spin to low spin transition of the iron in the heme cofactor . This suggests that L-Thiocitrulline contributes the sixth ligand to heme iron, probably through the thioureido sulfur .
Biochemical Pathways
The inhibition of NOS by L-Thiocitrulline affects the biochemical pathway that converts L-arginine to citrulline and nitric oxide (NO) . This pathway involves two successive monooxygenase reactions: the first is a ferryl heme-dependent hydroxylation of a guanidino nitrogen of arginine yielding NOH-Arg, and the second is a nucleophilic attack by peroxy heme on the guanidino carbon of NOH-Arg or on the one-electron oxidation product of NOH-Arg .
Pharmacokinetics
It is known that the compound’s binding to nos is reversible . More research is needed to fully understand the ADME (Absorption, Distribution, Metabolism, and Excretion) properties of L-Thiocitrulline and their impact on its bioavailability .
Result of Action
The inhibition of NOS by L-Thiocitrulline can reverse pathologies associated with the overproduction of NO, such as the hypotension of septic shock . The S-alkyl-L-thiocitrullines, a class of compounds that includes L-Thiocitrulline, have strong pressor activity in normotensive control rats; S-methyl-L-thiocitrulline reverses hypotension in a rat model of septic peritonitis and in dogs administered endotoxin .
Action Environment
It is known that the compound’s inhibitory action on nos is stereospecific , suggesting that its efficacy could be influenced by the stereochemical environment of the enzyme’s active site
Zukünftige Richtungen
Eigenschaften
IUPAC Name |
(2S)-2-amino-5-(carbamothioylamino)pentanoic acid | |
---|---|---|
Source | PubChem | |
URL | https://pubchem.ncbi.nlm.nih.gov | |
Description | Data deposited in or computed by PubChem | |
InChI |
InChI=1S/C6H13N3O2S/c7-4(5(10)11)2-1-3-9-6(8)12/h4H,1-3,7H2,(H,10,11)(H3,8,9,12)/t4-/m0/s1 | |
Source | PubChem | |
URL | https://pubchem.ncbi.nlm.nih.gov | |
Description | Data deposited in or computed by PubChem | |
InChI Key |
BKGWACHYAMTLAF-BYPYZUCNSA-N | |
Source | PubChem | |
URL | https://pubchem.ncbi.nlm.nih.gov | |
Description | Data deposited in or computed by PubChem | |
Canonical SMILES |
C(CC(C(=O)O)N)CNC(=S)N | |
Source | PubChem | |
URL | https://pubchem.ncbi.nlm.nih.gov | |
Description | Data deposited in or computed by PubChem | |
Isomeric SMILES |
C(C[C@@H](C(=O)O)N)CNC(=S)N | |
Source | PubChem | |
URL | https://pubchem.ncbi.nlm.nih.gov | |
Description | Data deposited in or computed by PubChem | |
Molecular Formula |
C6H13N3O2S | |
Source | PubChem | |
URL | https://pubchem.ncbi.nlm.nih.gov | |
Description | Data deposited in or computed by PubChem | |
Molecular Weight |
191.25 g/mol | |
Source | PubChem | |
URL | https://pubchem.ncbi.nlm.nih.gov | |
Description | Data deposited in or computed by PubChem | |
Product Name |
L-thiocitrulline | |
CAS RN |
156719-37-8 | |
Record name | Thiocitrulline | |
Source | DrugBank | |
URL | https://www.drugbank.ca/drugs/DB03953 | |
Description | The DrugBank database is a unique bioinformatics and cheminformatics resource that combines detailed drug (i.e. chemical, pharmacological and pharmaceutical) data with comprehensive drug target (i.e. sequence, structure, and pathway) information. | |
Explanation | Creative Common's Attribution-NonCommercial 4.0 International License (http://creativecommons.org/licenses/by-nc/4.0/legalcode) | |
Retrosynthesis Analysis
AI-Powered Synthesis Planning: Our tool employs the Template_relevance Pistachio, Template_relevance Bkms_metabolic, Template_relevance Pistachio_ringbreaker, Template_relevance Reaxys, Template_relevance Reaxys_biocatalysis model, leveraging a vast database of chemical reactions to predict feasible synthetic routes.
One-Step Synthesis Focus: Specifically designed for one-step synthesis, it provides concise and direct routes for your target compounds, streamlining the synthesis process.
Accurate Predictions: Utilizing the extensive PISTACHIO, BKMS_METABOLIC, PISTACHIO_RINGBREAKER, REAXYS, REAXYS_BIOCATALYSIS database, our tool offers high-accuracy predictions, reflecting the latest in chemical research and data.
Strategy Settings
Precursor scoring | Relevance Heuristic |
---|---|
Min. plausibility | 0.01 |
Model | Template_relevance |
Template Set | Pistachio/Bkms_metabolic/Pistachio_ringbreaker/Reaxys/Reaxys_biocatalysis |
Top-N result to add to graph | 6 |
Feasible Synthetic Routes
Haftungsausschluss und Informationen zu In-Vitro-Forschungsprodukten
Bitte beachten Sie, dass alle Artikel und Produktinformationen, die auf BenchChem präsentiert werden, ausschließlich zu Informationszwecken bestimmt sind. Die auf BenchChem zum Kauf angebotenen Produkte sind speziell für In-vitro-Studien konzipiert, die außerhalb lebender Organismen durchgeführt werden. In-vitro-Studien, abgeleitet von dem lateinischen Begriff "in Glas", beinhalten Experimente, die in kontrollierten Laborumgebungen unter Verwendung von Zellen oder Geweben durchgeführt werden. Es ist wichtig zu beachten, dass diese Produkte nicht als Arzneimittel oder Medikamente eingestuft sind und keine Zulassung der FDA für die Vorbeugung, Behandlung oder Heilung von medizinischen Zuständen, Beschwerden oder Krankheiten erhalten haben. Wir müssen betonen, dass jede Form der körperlichen Einführung dieser Produkte in Menschen oder Tiere gesetzlich strikt untersagt ist. Es ist unerlässlich, sich an diese Richtlinien zu halten, um die Einhaltung rechtlicher und ethischer Standards in Forschung und Experiment zu gewährleisten.