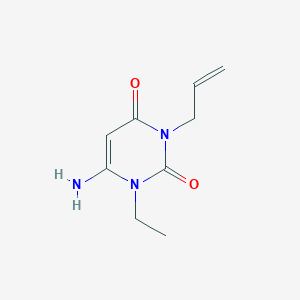
3-Allyl-6-amino-1-ethyluracil
Übersicht
Beschreibung
Gapromidin: ist eine synthetische Verbindung, die aufgrund ihrer einzigartigen chemischen Eigenschaften und möglichen Anwendungen in verschiedenen wissenschaftlichen Bereichen Interesse geweckt hat. Sie ist bekannt für ihre Stabilität und Reaktivität, was sie zu einem wertvollen Forschungsgegenstand in Chemie, Biologie, Medizin und Industrie macht.
Herstellungsmethoden
Synthesewege und Reaktionsbedingungen: Die Synthese von Gapromidin erfolgt in der Regel in einem mehrstufigen Verfahren, das die Bildung von Zwischenprodukten beinhaltet. Der erste Schritt umfasst häufig die Reaktion von primären Aminen mit bestimmten Aldehyden unter kontrollierten Bedingungen zur Bildung von Iminen. Diese Imine werden dann unter Verwendung von Hydrierungsverfahren zu den gewünschten Amin-Derivaten reduziert. Der letzte Schritt beinhaltet die Cyclisierung dieser Derivate unter sauren oder basischen Bedingungen zur Bildung von Gapromidin.
Industrielle Produktionsmethoden: Die industrielle Produktion von Gapromidin folgt einem ähnlichen Syntheseweg, jedoch in größerem Maßstab. Der Prozess ist auf Ausbeute und Reinheit optimiert und beinhaltet häufig kontinuierliche Durchflussreaktoren und automatisierte Systeme, um eine gleichbleibende Produktion zu gewährleisten. Die Verwendung von Hochdruckhydrierung und fortschrittlichen Reinigungsverfahren, wie z. B. Chromatographie, ist in industriellen Umgebungen üblich.
Chemische Reaktionsanalyse
Arten von Reaktionen: Gapromidin durchläuft verschiedene chemische Reaktionen, darunter:
Oxidation: Gapromidin kann mit starken Oxidationsmitteln wie Kaliumpermanganat oder Chromtrioxid oxidiert werden, was zur Bildung der entsprechenden Oxide führt.
Reduktion: Reduktionsreaktionen beinhalten die Verwendung von Reduktionsmitteln wie Lithiumaluminiumhydrid oder Natriumborhydrid, um Gapromidin in seine reduzierten Formen umzuwandeln.
Substitution: Nucleophile Substitutionsreaktionen können mit halogenierten Derivaten von Gapromidin auftreten, wobei Halogenatome durch andere Nucleophile ersetzt werden.
Häufige Reagenzien und Bedingungen:
Oxidation: Kaliumpermanganat, Chromtrioxid, saure oder basische Bedingungen.
Reduktion: Lithiumaluminiumhydrid, Natriumborhydrid, wasserfreie Bedingungen.
Substitution: Halogenierte Gapromidin-Derivate, Nucleophile wie Hydroxid-Ionen, unter Rückflussbedingungen.
Hauptprodukte:
Oxidation: Bildung von Oxiden und hydroxylierten Derivaten.
Reduktion: Bildung von Amin-Derivaten.
Substitution: Bildung von substituierten Gapromidin-Verbindungen mit verschiedenen funktionellen Gruppen.
Wissenschaftliche Forschungsanwendungen
Gapromidin hat eine breite Palette von Anwendungen in der wissenschaftlichen Forschung:
Chemie: Wird als Reagenz in der organischen Synthese und als Katalysator in verschiedenen chemischen Reaktionen verwendet.
Biologie: Wird auf sein Potenzial als Enzyminhibitor und seine Wechselwirkungen mit biologischen Makromolekülen untersucht.
Medizin: Wird auf seine potenziellen therapeutischen Wirkungen untersucht, darunter die Verwendung als Antikonvulsivum und bei der Behandlung von neurologischen Erkrankungen.
Industrie: Wird bei der Herstellung von Spezialchemikalien und als Zwischenprodukt bei der Synthese von Pharmazeutika eingesetzt.
Wirkmechanismus
Der Wirkmechanismus von Gapromidin beinhaltet seine Wechselwirkung mit spezifischen molekularen Zielstrukturen, wie z. B. spannungsgesteuerten Natriumkanälen und Kalziumkanälen. Durch die Veränderung des Transports von Natrium- und Kalziumionen über Zellmembranen reduziert Gapromidin die Frequenz der Nervenerregung, was für seine antikonvulsiven Wirkungen verantwortlich ist. Zusätzlich tragen seine Metaboliten, wie Phenobarbital und Phenylethylmalonamid, zu seiner gesamten pharmakologischen Aktivität bei .
Vorbereitungsmethoden
Synthetic Routes and Reaction Conditions: The synthesis of Gapromidine typically involves a multi-step process that includes the formation of intermediate compounds. The initial step often involves the reaction of primary amines with specific aldehydes under controlled conditions to form imines. These imines are then reduced using hydrogenation techniques to yield the desired amine derivatives. The final step involves the cyclization of these derivatives under acidic or basic conditions to form Gapromidine.
Industrial Production Methods: Industrial production of Gapromidine follows a similar synthetic route but on a larger scale. The process is optimized for yield and purity, often involving continuous flow reactors and automated systems to ensure consistent production. The use of high-pressure hydrogenation and advanced purification techniques, such as chromatography, is common in industrial settings.
Analyse Chemischer Reaktionen
Types of Reactions: Gapromidine undergoes various chemical reactions, including:
Oxidation: Gapromidine can be oxidized using strong oxidizing agents like potassium permanganate or chromium trioxide, leading to the formation of corresponding oxides.
Reduction: Reduction reactions involve the use of reducing agents such as lithium aluminum hydride or sodium borohydride to convert Gapromidine into its reduced forms.
Substitution: Nucleophilic substitution reactions can occur with halogenated derivatives of Gapromidine, where halogen atoms are replaced by other nucleophiles.
Common Reagents and Conditions:
Oxidation: Potassium permanganate, chromium trioxide, acidic or basic conditions.
Reduction: Lithium aluminum hydride, sodium borohydride, anhydrous conditions.
Substitution: Halogenated Gapromidine derivatives, nucleophiles like hydroxide ions, under reflux conditions.
Major Products:
Oxidation: Formation of oxides and hydroxylated derivatives.
Reduction: Formation of amine derivatives.
Substitution: Formation of substituted Gapromidine compounds with various functional groups.
Wissenschaftliche Forschungsanwendungen
Gapromidine has a wide range of applications in scientific research:
Chemistry: Used as a reagent in organic synthesis and as a catalyst in various chemical reactions.
Biology: Studied for its potential as an enzyme inhibitor and its interactions with biological macromolecules.
Medicine: Investigated for its potential therapeutic effects, including its use as an anticonvulsant and in the treatment of neurological disorders.
Industry: Utilized in the production of specialty chemicals and as an intermediate in the synthesis of pharmaceuticals.
Wirkmechanismus
The mechanism of action of Gapromidine involves its interaction with specific molecular targets, such as voltage-gated sodium channels and calcium channels. By altering the transport of sodium and calcium ions across cell membranes, Gapromidine reduces the frequency of nerve firing, which is responsible for its anticonvulsant effects. Additionally, its metabolites, such as phenobarbital and phenylethylmalonamide, contribute to its overall pharmacological activity .
Vergleich Mit ähnlichen Verbindungen
Gapromidin kann mit anderen ähnlichen Verbindungen verglichen werden, wie z. B.:
Primidon: Beide Verbindungen werden als Antikonvulsiva eingesetzt, jedoch hat Gapromidin einen anderen Stoffwechselweg und eine einzigartige Reihe von Metaboliten.
Phenobarbital: Während beide antikonvulsive Eigenschaften besitzen, beinhaltet der Wirkmechanismus von Gapromidin zusätzliche Wege und Metaboliten.
Phenylethylmalonamid: Ein Metabolit von Gapromidin, der einige pharmakologische Eigenschaften teilt, sich aber in seinen spezifischen Wechselwirkungen und Wirkungen unterscheidet.
Eigenschaften
IUPAC Name |
6-amino-1-ethyl-3-prop-2-enylpyrimidine-2,4-dione | |
---|---|---|
Source | PubChem | |
URL | https://pubchem.ncbi.nlm.nih.gov | |
Description | Data deposited in or computed by PubChem | |
InChI |
InChI=1S/C9H13N3O2/c1-3-5-12-8(13)6-7(10)11(4-2)9(12)14/h3,6H,1,4-5,10H2,2H3 | |
Source | PubChem | |
URL | https://pubchem.ncbi.nlm.nih.gov | |
Description | Data deposited in or computed by PubChem | |
InChI Key |
VQHXZPNWCYIWNV-UHFFFAOYSA-N | |
Source | PubChem | |
URL | https://pubchem.ncbi.nlm.nih.gov | |
Description | Data deposited in or computed by PubChem | |
Canonical SMILES |
CCN1C(=CC(=O)N(C1=O)CC=C)N | |
Source | PubChem | |
URL | https://pubchem.ncbi.nlm.nih.gov | |
Description | Data deposited in or computed by PubChem | |
Molecular Formula |
C9H13N3O2 | |
Source | PubChem | |
URL | https://pubchem.ncbi.nlm.nih.gov | |
Description | Data deposited in or computed by PubChem | |
DSSTOX Substance ID |
DTXSID90222678 | |
Record name | Uracil, 1-allyl-6-amino-3-ethyl- mixed with 3-allyl-6-amino-1-ethyluracil (4:1) | |
Source | EPA DSSTox | |
URL | https://comptox.epa.gov/dashboard/DTXSID90222678 | |
Description | DSSTox provides a high quality public chemistry resource for supporting improved predictive toxicology. | |
Molecular Weight |
195.22 g/mol | |
Source | PubChem | |
URL | https://pubchem.ncbi.nlm.nih.gov | |
Description | Data deposited in or computed by PubChem | |
CAS No. |
72361-29-6 | |
Record name | Uracil, 1-allyl-6-amino-3-ethyl- mixed with 3-allyl-6-amino-1-ethyluracil (4:1) | |
Source | ChemIDplus | |
URL | https://pubchem.ncbi.nlm.nih.gov/substance/?source=chemidplus&sourceid=0072361296 | |
Description | ChemIDplus is a free, web search system that provides access to the structure and nomenclature authority files used for the identification of chemical substances cited in National Library of Medicine (NLM) databases, including the TOXNET system. | |
Record name | Uracil, 1-allyl-6-amino-3-ethyl- mixed with 3-allyl-6-amino-1-ethyluracil (4:1) | |
Source | EPA DSSTox | |
URL | https://comptox.epa.gov/dashboard/DTXSID90222678 | |
Description | DSSTox provides a high quality public chemistry resource for supporting improved predictive toxicology. | |
Haftungsausschluss und Informationen zu In-Vitro-Forschungsprodukten
Bitte beachten Sie, dass alle Artikel und Produktinformationen, die auf BenchChem präsentiert werden, ausschließlich zu Informationszwecken bestimmt sind. Die auf BenchChem zum Kauf angebotenen Produkte sind speziell für In-vitro-Studien konzipiert, die außerhalb lebender Organismen durchgeführt werden. In-vitro-Studien, abgeleitet von dem lateinischen Begriff "in Glas", beinhalten Experimente, die in kontrollierten Laborumgebungen unter Verwendung von Zellen oder Geweben durchgeführt werden. Es ist wichtig zu beachten, dass diese Produkte nicht als Arzneimittel oder Medikamente eingestuft sind und keine Zulassung der FDA für die Vorbeugung, Behandlung oder Heilung von medizinischen Zuständen, Beschwerden oder Krankheiten erhalten haben. Wir müssen betonen, dass jede Form der körperlichen Einführung dieser Produkte in Menschen oder Tiere gesetzlich strikt untersagt ist. Es ist unerlässlich, sich an diese Richtlinien zu halten, um die Einhaltung rechtlicher und ethischer Standards in Forschung und Experiment zu gewährleisten.