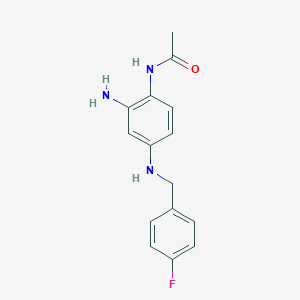
N-Acetyl Retigabine
Übersicht
Beschreibung
N-Acetyl Retigabine (CAS: 229970-68-7; molecular formula: C₁₅H₁₆FN₃O) is the primary acetylated metabolite of the anticonvulsant drug retigabine (ezogabine), which is approved for adjunctive treatment of partial-onset seizures in adults . Retigabine itself is metabolized extensively via N-glucuronidation and acetylation, with this compound accounting for a significant portion of circulating metabolites. Unlike cytochrome P450-mediated pathways, retigabine’s metabolism is dominated by these Phase II reactions, leading to high urinary excretion of the parent drug and its metabolites . This compound is chemically stable under controlled conditions (storage at +4°C, transport at room temperature) and has a purity exceeding 95% (HPLC) in commercial preparations .
Vorbereitungsmethoden
Synthetic Routes and Reaction Conditions
The synthesis of N-Acetyl Retigabine typically involves the reaction of 4-fluorobenzylamine with 2-amino-4-nitrophenylacetamide under reductive conditions. The nitro group is reduced to an amino group using a suitable reducing agent such as palladium on carbon (Pd/C) in the presence of hydrogen gas. The reaction is carried out in an appropriate solvent, such as ethanol or methanol, at elevated temperatures to facilitate the reduction process .
Industrial Production Methods
Industrial production of this compound may involve similar synthetic routes but on a larger scale. The use of continuous flow reactors and automated systems can enhance the efficiency and yield of the synthesis. Additionally, purification techniques such as recrystallization and chromatography are employed to obtain the desired product with high purity .
Analyse Chemischer Reaktionen
Types of Reactions
N-Acetyl Retigabine undergoes various chemical reactions, including:
Oxidation: The amino groups can be oxidized to form nitroso or nitro derivatives.
Reduction: The nitro group in the precursor can be reduced to an amino group.
Substitution: The fluorine atom on the phenyl ring can be substituted with other nucleophiles under appropriate conditions.
Common Reagents and Conditions
Oxidation: Common oxidizing agents include potassium permanganate (KMnO₄) and hydrogen peroxide (H₂O₂).
Reduction: Palladium on carbon (Pd/C) and hydrogen gas are commonly used for the reduction of nitro groups.
Substitution: Nucleophiles such as amines, thiols, and alkoxides can be used for substitution reactions.
Major Products Formed
Oxidation: Formation of nitroso or nitro derivatives.
Reduction: Conversion of nitro groups to amino groups.
Substitution: Formation of substituted phenyl derivatives.
Wissenschaftliche Forschungsanwendungen
Research Applications
-
Anticonvulsant Activity
- Preclinical Studies : N-Acetyl Retigabine has shown anticonvulsant properties in animal models. Although it is approximately 20 times less potent than its parent compound, Retigabine, it still exhibits efficacy in reducing seizure activity .
- Case Studies : Initial studies indicate that NAMR can mitigate seizure frequency and intensity in models of electrically and chemically induced seizures .
- Neuropathic Pain Management
- Anxiolytic Effects
Pharmacokinetics and Safety Profile
This compound is absorbed rapidly and exhibits good tolerability in early clinical trials. The pharmacokinetic profile indicates that it is resistant to first-pass metabolism, which enhances its bioavailability . Adverse effects reported include mild dizziness and somnolence, but no significant issues related to tolerance or dependence have been noted .
Clinical Trials and Future Directions
Currently, clinical research on this compound is limited but promising. Ongoing studies aim to assess its safety and efficacy as adjunctive therapy for uncontrolled partial-onset seizures in adolescents and adults . The compound's potential interactions with other medications, such as digoxin, are also under investigation, highlighting the importance of understanding its pharmacodynamics .
Wirkmechanismus
The mechanism of action of N-Acetyl Retigabine involves its interaction with specific molecular targets, such as enzymes and receptors. The compound can inhibit the activity of certain enzymes by binding to their active sites, thereby blocking substrate access. Additionally, it can interact with cellular receptors, modulating signal transduction pathways and affecting cellular functions .
Vergleich Mit ähnlichen Verbindungen
Comparison with Structural Analogues
Table 1: Structural and Pharmacokinetic Comparison
*Estimated based on molecular formulas in .
Polymorphic Forms of Retigabine
Retigabine’s polymorphs (e.g., Forms A, B, C) exhibit varying stability and solubility. Novel polymorphs developed by SYMED LABS claim superior crystallinity and chemical stability, addressing limitations of earlier forms . In contrast, this compound’s stability is influenced by its resistance to enzymatic hydrolysis, unlike labile N-glucuronide metabolites of retigabine, which degrade under suboptimal pH or temperature, artificially inflating plasma concentrations of the parent drug and this compound during analysis .
Pharmacodynamic Comparisons
Mechanism of Action
Retigabine and this compound both act on neuronal potassium channels, but with differing selectivity:
- This compound : Contributes to prolonged anticonvulsant effects as an active metabolite but with reduced potency compared to retigabine .
- ARA-S (Arachidonoyl-L-serine): An endocannabinoid tested in combination with retigabine, showing complementary KV7 subtype selectivity (e.g., KV7.4/7.5) and reduced off-target effects .
Table 2: Pharmacodynamic Profiles
*Assumed based on structural similarity.
Synergistic Combinations
Co-administration of retigabine with ARA-S at low doses enhances M-channel activation while minimizing adverse effects, demonstrating a 2.5-fold increase in therapeutic index compared to retigabine monotherapy .
Clinical and Pharmacokinetic Considerations
Biologische Aktivität
N-Acetyl Retigabine, a metabolite of the antiepileptic drug Retigabine (also known as Ezogabine), has garnered attention due to its potential biological activities, particularly in the context of neuronal excitability and seizure management. This article provides a comprehensive overview of the biological activity of this compound, including pharmacokinetics, mechanisms of action, and relevant case studies.
Overview of Retigabine and Its Metabolites
Retigabine is a first-in-class antiepileptic drug that enhances the activity of KCNQ (K(v)7) potassium channels, thereby reducing neuronal excitability. It is primarily used as an adjunctive therapy for adults with partial-onset seizures. Upon administration, Retigabine undergoes extensive metabolism, primarily through N-acetylation and N-glucuronidation, leading to the formation of its N-acetyl metabolite (this compound) .
Pharmacokinetics of this compound
The pharmacokinetic profile of this compound is crucial for understanding its therapeutic potential. Studies indicate that:
- Absorption : Retigabine is rapidly absorbed, with peak plasma concentrations achieved within 0.5 to 2 hours post-administration.
- Half-Life : The elimination half-life of Retigabine ranges from 6 to 8 hours in adults, whereas the half-life for this compound has not been explicitly detailed but is expected to be influenced by the parent compound's pharmacokinetics .
- Bioavailability : The absolute oral bioavailability of Retigabine is approximately 60%, suggesting that this compound may also exhibit significant bioavailability .
This compound acts primarily by enhancing KCNQ potassium channel activity. This modulation leads to hyperpolarization of neuronal membranes, resulting in decreased neuronal excitability and reduced seizure activity. The specific interaction with KCNQ channels positions it as a critical player in managing epilepsy and other hyperexcitability disorders .
Anticonvulsant Efficacy
This compound has demonstrated anticonvulsant properties in various preclinical models. For example:
- In Vivo Studies : In animal models, retigabine has shown efficacy against generalized tonic-clonic seizures and partial seizures induced by various stimuli . Repeated administration has been linked to increased neuronal apoptosis in certain brain regions, suggesting a complex relationship between dosage, exposure duration, and biological effects .
Neuroprotective Effects
Research indicates that retigabine may possess neuroprotective properties beyond its anticonvulsant effects. The modulation of KCNQ channels may help protect against excitotoxicity associated with seizures and other neurological conditions .
Case Studies and Research Findings
Several studies have explored the biological activity of this compound:
- Neuronal Apoptosis Study : A study investigated the effects of retigabine on neuronal apoptosis in neonatal rats. Acute exposure did not induce apoptosis; however, repeated doses led to significant increases in apoptosis in specific brain regions .
- Pharmacokinetic Analysis : A clinical study assessed the pharmacokinetics and safety profile of retigabine in adolescents with uncontrolled partial-onset seizures. The study highlighted the systemic exposure levels of this compound during treatment .
- Quantitative Method Development : Research focused on developing a quantitative method for accurately measuring retigabine and its metabolites in human plasma. This work is essential for understanding the pharmacokinetics and dynamics of this compound in clinical settings .
Summary Table of Key Findings
Study Focus | Findings |
---|---|
Pharmacokinetics | Rapid absorption; half-life ~6-8 hours; bioavailability ~60% |
Mechanism | Enhances KCNQ channel activity; reduces neuronal excitability |
Anticonvulsant Efficacy | Effective against various seizure types; repeated doses linked to increased apoptosis |
Neuroprotective Effects | Potential protective effects against excitotoxicity |
Q & A
Basic Research Questions
Q. How is N-Acetyl Retigabine synthesized and characterized in preclinical research?
this compound is synthesized via acetylation of the parent compound Retigabine (Ezogabine). Key steps include:
- Chemical modification : Retigabine’s amino group undergoes acetylation using acetic anhydride or acetyl chloride under controlled conditions .
- Characterization : Purity (>95%) is validated via HPLC, with structural confirmation using NMR and mass spectrometry (Exact Mass: 273.1277; SMILES: CC(=O)Nc1ccc(NCc2ccc(F)cc2)cc1N) .
- Storage : Stabilize at +4°C to prevent degradation, with transport at room temperature .
Q. What analytical techniques are essential for assessing this compound’s purity and stability?
- High-Performance Liquid Chromatography (HPLC) : Quantifies purity (>95%) and detects degradation products .
- Mass Spectrometry (MS) : Confirms molecular weight (273.31 g/mol) and fragmentation patterns .
- Nuclear Magnetic Resonance (NMR) : Validates structural integrity, particularly the acetyl group’s position .
- Stability testing : Conduct accelerated degradation studies under varying pH, temperature, and light conditions to establish shelf-life .
Q. What is the hypothesized mechanism of action of this compound as a neuronal potassium channel modulator?
this compound, like its parent compound, activates KCNQ (Kv7) potassium channels, inducing neuronal hyperpolarization and reducing excitability. Key evidence includes:
- In vitro electrophysiology : Patch-clamp studies demonstrating increased K+ currents in transfected HEK293 cells .
- Metabolite activity : As the primary metabolite of Retigabine (NAMR), it contributes to sustained anticonvulsant effects by prolonging channel opening .
Advanced Research Questions
Q. How do methodological approaches differ when evaluating this compound’s efficacy in in vivo vs. in vitro models?
- In vitro models : Use transfected cell lines (e.g., HEK293) to isolate KCNQ channel activity. Measure dose-response curves (e.g., EC50) via automated patch-clamp systems .
- In vivo models : Employ rodent seizure models (e.g., maximal electroshock or kainate-induced seizures). Monitor plasma concentrations to correlate efficacy with pharmacokinetics (e.g., Cmax, half-life) .
- Key challenge : Address discrepancies between in vitro potency and in vivo bioavailability due to metabolite conversion (e.g., NAMR’s role) .
Q. What experimental strategies resolve contradictions in this compound’s pharmacokinetic and pharmacodynamic profiles?
- Metabolite profiling : Use LC-MS/MS to quantify this compound and its secondary metabolites in plasma and cerebrospinal fluid .
- Tissue distribution studies : Radiolabeled compounds (e.g., ¹⁴C) track penetration across the blood-brain barrier .
- Dynamic clamp electrophysiology : Simulate channel behavior in neurons to disentangle direct vs. metabolite-mediated effects .
Q. What are the methodological limitations in studying this compound’s long-term neuroprotective effects?
- Chronic toxicity models : Rodent studies require ≥6-month dosing to assess hepatotoxicity or CNS side effects (e.g., sedation) .
- Biomarker selection : Validate surrogate markers (e.g., glial fibrillary acidic protein for neuroinflammation) to reduce reliance on histopathology .
- Data interpretation : Differentiate drug-induced effects from compensatory mechanisms (e.g., upregulated KCNQ expression) via transcriptomic analysis .
Q. How can researchers optimize experimental design for studying this compound’s interactions with co-administered anticonvulsants?
- Isobolographic analysis : Quantify synergistic or antagonistic interactions with drugs like valproate or levetiracetam .
- Microdialysis in freely moving animals : Monitor extracellular drug concentrations in real time to model pharmacokinetic interactions .
- Computational modeling : Use tools like PK-Sim to predict dose adjustments and avoid therapeutic failures .
Q. Methodological Best Practices
- Reproducibility : Document synthesis protocols, storage conditions, and analytical parameters in line with ICH guidelines .
- Data reporting : Include raw chromatograms, electrophysiological traces, and statistical tests (e.g., ANOVA with post-hoc corrections) to support conclusions .
- Ethical compliance : Adhere to animal welfare standards (e.g., ARRIVE guidelines) in in vivo studies .
Eigenschaften
IUPAC Name |
N-[2-amino-4-[(4-fluorophenyl)methylamino]phenyl]acetamide | |
---|---|---|
Source | PubChem | |
URL | https://pubchem.ncbi.nlm.nih.gov | |
Description | Data deposited in or computed by PubChem | |
InChI |
InChI=1S/C15H16FN3O/c1-10(20)19-15-7-6-13(8-14(15)17)18-9-11-2-4-12(16)5-3-11/h2-8,18H,9,17H2,1H3,(H,19,20) | |
Source | PubChem | |
URL | https://pubchem.ncbi.nlm.nih.gov | |
Description | Data deposited in or computed by PubChem | |
InChI Key |
YGNSHYRMOVKKSL-UHFFFAOYSA-N | |
Source | PubChem | |
URL | https://pubchem.ncbi.nlm.nih.gov | |
Description | Data deposited in or computed by PubChem | |
Canonical SMILES |
CC(=O)NC1=C(C=C(C=C1)NCC2=CC=C(C=C2)F)N | |
Source | PubChem | |
URL | https://pubchem.ncbi.nlm.nih.gov | |
Description | Data deposited in or computed by PubChem | |
Molecular Formula |
C15H16FN3O | |
Source | PubChem | |
URL | https://pubchem.ncbi.nlm.nih.gov | |
Description | Data deposited in or computed by PubChem | |
Molecular Weight |
273.30 g/mol | |
Source | PubChem | |
URL | https://pubchem.ncbi.nlm.nih.gov | |
Description | Data deposited in or computed by PubChem | |
CAS No. |
229970-68-7 | |
Record name | 229970-68-7 | |
Source | European Chemicals Agency (ECHA) | |
URL | https://echa.europa.eu/information-on-chemicals | |
Description | The European Chemicals Agency (ECHA) is an agency of the European Union which is the driving force among regulatory authorities in implementing the EU's groundbreaking chemicals legislation for the benefit of human health and the environment as well as for innovation and competitiveness. | |
Explanation | Use of the information, documents and data from the ECHA website is subject to the terms and conditions of this Legal Notice, and subject to other binding limitations provided for under applicable law, the information, documents and data made available on the ECHA website may be reproduced, distributed and/or used, totally or in part, for non-commercial purposes provided that ECHA is acknowledged as the source: "Source: European Chemicals Agency, http://echa.europa.eu/". Such acknowledgement must be included in each copy of the material. ECHA permits and encourages organisations and individuals to create links to the ECHA website under the following cumulative conditions: Links can only be made to webpages that provide a link to the Legal Notice page. | |
Haftungsausschluss und Informationen zu In-Vitro-Forschungsprodukten
Bitte beachten Sie, dass alle Artikel und Produktinformationen, die auf BenchChem präsentiert werden, ausschließlich zu Informationszwecken bestimmt sind. Die auf BenchChem zum Kauf angebotenen Produkte sind speziell für In-vitro-Studien konzipiert, die außerhalb lebender Organismen durchgeführt werden. In-vitro-Studien, abgeleitet von dem lateinischen Begriff "in Glas", beinhalten Experimente, die in kontrollierten Laborumgebungen unter Verwendung von Zellen oder Geweben durchgeführt werden. Es ist wichtig zu beachten, dass diese Produkte nicht als Arzneimittel oder Medikamente eingestuft sind und keine Zulassung der FDA für die Vorbeugung, Behandlung oder Heilung von medizinischen Zuständen, Beschwerden oder Krankheiten erhalten haben. Wir müssen betonen, dass jede Form der körperlichen Einführung dieser Produkte in Menschen oder Tiere gesetzlich strikt untersagt ist. Es ist unerlässlich, sich an diese Richtlinien zu halten, um die Einhaltung rechtlicher und ethischer Standards in Forschung und Experiment zu gewährleisten.