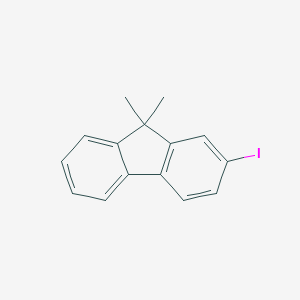
2-Iod-9,9-Dimethyl-9H-Fluoren
Übersicht
Beschreibung
2-Iodo-9,9-dimethyl-9H-fluorene is an organic compound with the chemical formula C15H13I. It is an organic halide and appears as a white to pale yellow crystalline substance . This compound is primarily used in organic synthesis, particularly as a starting material for the synthesis of aromatic compounds .
Wissenschaftliche Forschungsanwendungen
2-Iodo-9,9-dimethyl-9H-fluorene has several scientific research applications:
Organic Synthesis: It is used as a starting material for synthesizing various aromatic compounds, including fluorescent dyes, drugs, and polymer materials.
Material Science: This compound is utilized in the development of organic electronic materials, such as organic light-emitting diodes (OLEDs) and organic solar cells (OSCs).
Biological Research: It can be used in the synthesis of biologically active molecules for medicinal chemistry research.
Wirkmechanismus
Pharmacokinetics
It is a substrate for P-gp and an inhibitor for CYP1A2 and CYP2C19 . Its lipophilicity (Log Po/w) is 3.04 (iLOGP) and 5.07 (XLOGP3) .
Action Environment
The action of 2-Iodo-9,9-dimethyl-9H-fluorene can be influenced by environmental factors such as temperature and light. It is recommended to be stored in a dark place, sealed in dry conditions, at room temperature . These conditions likely help maintain the stability and efficacy of the compound.
Vorbereitungsmethoden
The preparation of 2-iodo-9,9-dimethyl-9H-fluorene can be achieved through the iodination of 2-fluorenyl-9,9-dimethylfluorene. A common method involves reacting 2-fluorenyl-9,9-dimethylfluorene with iodide in a suitable solvent . The reaction conditions typically include maintaining the reaction mixture at a specific temperature and using a catalyst to facilitate the iodination process .
Analyse Chemischer Reaktionen
2-Iodo-9,9-dimethyl-9H-fluorene undergoes various types of chemical reactions, including:
Substitution Reactions: This compound can participate in nucleophilic substitution reactions where the iodine atom is replaced by other nucleophiles.
Oxidation Reactions: It can be oxidized to form different products depending on the reagents and conditions used.
Reduction Reactions: Reduction of 2-iodo-9,9-dimethyl-9H-fluorene can lead to the formation of 9,9-dimethylfluorene.
Common reagents used in these reactions include halides, oxidizing agents, and reducing agents. The major products formed from these reactions vary based on the specific conditions and reagents employed .
Vergleich Mit ähnlichen Verbindungen
2-Iodo-9,9-dimethyl-9H-fluorene can be compared with other similar compounds, such as:
2-Bromo-9,9-dimethylfluorene: This compound has a bromine atom instead of an iodine atom, which affects its reactivity and applications.
2,7-Diiodo-9,9-dimethyl-9H-fluorene:
2-Iodo-9H-fluorene: This compound lacks the dimethyl groups, which can influence its chemical properties and uses.
The uniqueness of 2-iodo-9,9-dimethyl-9H-fluorene lies in its specific structure, which provides distinct reactivity and applications in various fields of research and industry .
Biologische Aktivität
2-Iodo-9,9-dimethyl-9H-fluorene is a derivative of fluorenes, which are a class of polycyclic aromatic hydrocarbons. This compound has garnered attention in recent years due to its potential biological activities, particularly in medicinal chemistry and material science. This article explores the biological activity of 2-iodo-9,9-dimethyl-9H-fluorene, highlighting its mechanisms of action, potential therapeutic applications, and relevant research findings.
- Molecular Formula : C13H11I
- Molecular Weight : 292.13 g/mol
- CAS Number : 333432-28-3
The biological activity of 2-iodo-9,9-dimethyl-9H-fluorene is primarily attributed to its ability to interact with various biomolecules. The presence of the iodine atom enhances the compound's electrophilicity, allowing it to participate in nucleophilic substitution reactions with biological targets such as proteins and nucleic acids.
Key Mechanisms:
- Receptor Binding : The compound may act as a ligand for specific receptors, modulating their activity.
- Enzyme Inhibition : It has been shown to inhibit certain enzymes involved in metabolic pathways, which can lead to altered cellular functions.
- Reactive Oxygen Species (ROS) Generation : Studies indicate that this compound can induce oxidative stress in cells by generating ROS, which can lead to apoptosis or necrosis.
Anticancer Properties
Research has indicated that 2-iodo-9,9-dimethyl-9H-fluorene exhibits significant anticancer activity. In vitro studies have demonstrated its effectiveness against various cancer cell lines, including breast and lung cancer cells. The compound's mechanism involves inducing cell cycle arrest and promoting apoptosis through the activation of caspase pathways.
Antimicrobial Activity
The compound has also shown promising antimicrobial properties against a range of bacterial strains. Its efficacy is thought to stem from its ability to disrupt bacterial cell membranes and inhibit key metabolic processes.
Case Studies
- Anticancer Study : A study published in Molecules demonstrated that 2-iodo-9,9-dimethyl-9H-fluorene significantly reduced the viability of MCF-7 breast cancer cells with an IC50 value of 15 µM. The study highlighted the compound's ability to induce apoptosis via the intrinsic pathway by upregulating pro-apoptotic proteins and downregulating anti-apoptotic proteins.
- Antimicrobial Efficacy : In a separate investigation focusing on antimicrobial properties, 2-iodo-9,9-dimethyl-9H-fluorene exhibited inhibitory effects against Staphylococcus aureus and Escherichia coli with minimum inhibitory concentrations (MICs) of 32 µg/mL and 64 µg/mL respectively .
Data Table
Eigenschaften
IUPAC Name |
2-iodo-9,9-dimethylfluorene | |
---|---|---|
Source | PubChem | |
URL | https://pubchem.ncbi.nlm.nih.gov | |
Description | Data deposited in or computed by PubChem | |
InChI |
InChI=1S/C15H13I/c1-15(2)13-6-4-3-5-11(13)12-8-7-10(16)9-14(12)15/h3-9H,1-2H3 | |
Source | PubChem | |
URL | https://pubchem.ncbi.nlm.nih.gov | |
Description | Data deposited in or computed by PubChem | |
InChI Key |
DVLSJPCXPNKPRJ-UHFFFAOYSA-N | |
Source | PubChem | |
URL | https://pubchem.ncbi.nlm.nih.gov | |
Description | Data deposited in or computed by PubChem | |
Canonical SMILES |
CC1(C2=CC=CC=C2C3=C1C=C(C=C3)I)C | |
Source | PubChem | |
URL | https://pubchem.ncbi.nlm.nih.gov | |
Description | Data deposited in or computed by PubChem | |
Molecular Formula |
C15H13I | |
Source | PubChem | |
URL | https://pubchem.ncbi.nlm.nih.gov | |
Description | Data deposited in or computed by PubChem | |
DSSTOX Substance ID |
DTXSID80452321 | |
Record name | 2-iodo-9,9-dimethyl-9H-fluorene | |
Source | EPA DSSTox | |
URL | https://comptox.epa.gov/dashboard/DTXSID80452321 | |
Description | DSSTox provides a high quality public chemistry resource for supporting improved predictive toxicology. | |
Molecular Weight |
320.17 g/mol | |
Source | PubChem | |
URL | https://pubchem.ncbi.nlm.nih.gov | |
Description | Data deposited in or computed by PubChem | |
CAS No. |
144981-85-1 | |
Record name | 2-iodo-9,9-dimethyl-9H-fluorene | |
Source | EPA DSSTox | |
URL | https://comptox.epa.gov/dashboard/DTXSID80452321 | |
Description | DSSTox provides a high quality public chemistry resource for supporting improved predictive toxicology. | |
Synthesis routes and methods I
Procedure details
Synthesis routes and methods II
Procedure details
Retrosynthesis Analysis
AI-Powered Synthesis Planning: Our tool employs the Template_relevance Pistachio, Template_relevance Bkms_metabolic, Template_relevance Pistachio_ringbreaker, Template_relevance Reaxys, Template_relevance Reaxys_biocatalysis model, leveraging a vast database of chemical reactions to predict feasible synthetic routes.
One-Step Synthesis Focus: Specifically designed for one-step synthesis, it provides concise and direct routes for your target compounds, streamlining the synthesis process.
Accurate Predictions: Utilizing the extensive PISTACHIO, BKMS_METABOLIC, PISTACHIO_RINGBREAKER, REAXYS, REAXYS_BIOCATALYSIS database, our tool offers high-accuracy predictions, reflecting the latest in chemical research and data.
Strategy Settings
Precursor scoring | Relevance Heuristic |
---|---|
Min. plausibility | 0.01 |
Model | Template_relevance |
Template Set | Pistachio/Bkms_metabolic/Pistachio_ringbreaker/Reaxys/Reaxys_biocatalysis |
Top-N result to add to graph | 6 |
Feasible Synthetic Routes
Haftungsausschluss und Informationen zu In-Vitro-Forschungsprodukten
Bitte beachten Sie, dass alle Artikel und Produktinformationen, die auf BenchChem präsentiert werden, ausschließlich zu Informationszwecken bestimmt sind. Die auf BenchChem zum Kauf angebotenen Produkte sind speziell für In-vitro-Studien konzipiert, die außerhalb lebender Organismen durchgeführt werden. In-vitro-Studien, abgeleitet von dem lateinischen Begriff "in Glas", beinhalten Experimente, die in kontrollierten Laborumgebungen unter Verwendung von Zellen oder Geweben durchgeführt werden. Es ist wichtig zu beachten, dass diese Produkte nicht als Arzneimittel oder Medikamente eingestuft sind und keine Zulassung der FDA für die Vorbeugung, Behandlung oder Heilung von medizinischen Zuständen, Beschwerden oder Krankheiten erhalten haben. Wir müssen betonen, dass jede Form der körperlichen Einführung dieser Produkte in Menschen oder Tiere gesetzlich strikt untersagt ist. Es ist unerlässlich, sich an diese Richtlinien zu halten, um die Einhaltung rechtlicher und ethischer Standards in Forschung und Experiment zu gewährleisten.