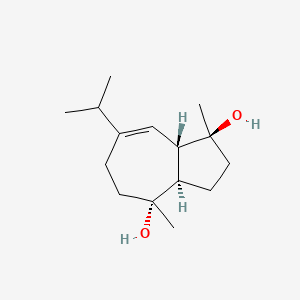
Alismoxid
Übersicht
Beschreibung
Alismoxid ist eine natürlich vorkommende Sesquiterpenoidverbindung, die aus den Rhizomen von Alisma orientale isoliert wird, einer Pflanze, die in der traditionellen Medizin häufig verwendet wird. Die Verbindung ist bekannt für ihre einzigartige chemische Struktur und verschiedene biologische Aktivitäten, darunter antibakterielle und entzündungshemmende Eigenschaften .
2. Herstellungsmethoden
Synthetische Wege und Reaktionsbedingungen: this compound kann durch verschiedene chemische Reaktionen synthetisiert werden. Eine gängige Methode beinhaltet die Extraktion der Verbindung aus den Rhizomen von Alisma orientale unter Verwendung organischer Lösungsmittel wie Aceton. Der Extrakt wird dann chromatographischen Verfahren unterzogen, um this compound zu isolieren .
Industrielle Produktionsmethoden: Die industrielle Produktion von this compound beinhaltet typischerweise die großtechnische Extraktion aus pflanzlichen Quellen. Der Prozess umfasst:
- Ernte und Trocknung der Rhizome von Alisma orientale.
- Mahlen der getrockneten Rhizome zu einem feinen Pulver.
- Extraktion der Verbindung mit Lösungsmitteln wie Aceton oder Ethanol.
- Reinigung des Extrakts durch Chromatographie, um reines this compound zu erhalten .
Wissenschaftliche Forschungsanwendungen
Alismoxid hat eine breite Palette von Anwendungen in der wissenschaftlichen Forschung:
Chemie: Wird als Vorläufer für die Synthese anderer komplexer Moleküle verwendet.
5. Wirkmechanismus
This compound übt seine Wirkungen über verschiedene molekulare Ziele und Wege aus:
Enzyminhibition: Es hemmt Enzyme wie Arginase, die eine Rolle im Harnstoffzyklus und der Stickstoffoxidregulierung spielen.
Entzündungshemmende Wege: This compound moduliert entzündungshemmende Wege durch Hemmung der Produktion von proinflammatorischen Zytokinen.
Antibakterielle Aktivität: Es stört bakterielle Zellmembranen, was zu Zelllyse und Zelltod führt.
Ähnliche Verbindungen:
- Alisol B 23-Acetat
- Alisol C 23-Acetat
- Alismol
- 10-O-Methyl-Alismoxid
- 11-Deoxyalisol C
Vergleich: this compound ist aufgrund seiner spezifischen Sesquiterpenoidstruktur einzigartig, die ihm unterschiedliche biologische Aktivitäten verleiht. Im Vergleich zu ähnlichen Verbindungen wie Alisol B 23-Acetat und Alisol C 23-Acetat weist this compound stärkere antibakterielle und entzündungshemmende Eigenschaften auf .
Wirkmechanismus
Target of Action
Alismoxide, a natural product, has been found to exhibit selective and potent competitive inhibition for human arginase I . Arginases are bimanganese enzymes that catalyze the hydrolysis of L-arginine to form L-ornithine and urea, playing a key role in the urea cycle and the regulation of nitric oxide (NO) homeostasis in mammals .
Mode of Action
The mode of action of Alismoxide involves its interaction with its primary target, human arginase I. Alismoxide acts as a competitive inhibitor, binding to the active site of the enzyme and preventing its normal substrate, L-arginine, from binding . This inhibitory effect disrupts the normal function of arginase I, altering the urea cycle and nitric oxide homeostasis .
Biochemical Pathways
The primary biochemical pathway affected by Alismoxide is the urea cycle, specifically the conversion of L-arginine to L-ornithine and urea. By inhibiting arginase I, Alismoxide disrupts this conversion, potentially leading to an accumulation of L-arginine and a decrease in L-ornithine and urea . This disruption can also affect nitric oxide homeostasis, as L-arginine is a precursor for nitric oxide synthesis .
Pharmacokinetics
They influence how much of the compound enters the systemic circulation, how it is distributed throughout the body, how it is metabolized, and how it is eliminated
Action Environment
It is known that environmental conditions can significantly impact the activity of various compounds Factors such as temperature, humidity, and pH can affect the stability, solubility, and bioavailability of a compound, potentially influencing its pharmacokinetic properties and overall effectiveness
Biochemische Analyse
Biochemical Properties
Alismoxide plays a significant role in biochemical reactions, particularly in its interactions with enzymes and proteins. It has been identified as a potent inhibitor of human arginase I, an enzyme involved in the urea cycle and nitric oxide regulation . Alismoxide exhibits competitive inhibition, binding to the active site of arginase I and preventing its activity. Additionally, alismoxide interacts with nuclear factor kappa B (NF-κB), inhibiting its activation and subsequently reducing the expression of inducible nitric oxide synthase (iNOS) .
Cellular Effects
Alismoxide exerts various effects on different cell types and cellular processes. In immune cells, it has been shown to reduce inflammation by inhibiting NF-κB activation and iNOS expression . This leads to decreased production of pro-inflammatory cytokines and nitric oxide. In airway epithelial cells, alismoxide alleviates airway hyperresponsiveness and remodeling, making it a potential therapeutic agent for asthma . Furthermore, alismoxide influences cell signaling pathways, gene expression, and cellular metabolism by modulating the activity of key enzymes and transcription factors.
Molecular Mechanism
The molecular mechanism of alismoxide involves its interaction with specific biomolecules and enzymes. Alismoxide binds to the active site of human arginase I, inhibiting its activity and reducing the production of urea and ornithine . This inhibition leads to increased levels of nitric oxide, which has various physiological effects, including vasodilation and immune modulation. Additionally, alismoxide inhibits NF-κB activation by preventing its translocation to the nucleus, thereby reducing the expression of pro-inflammatory genes .
Temporal Effects in Laboratory Settings
In laboratory settings, the effects of alismoxide have been observed to change over time. Alismoxide is relatively stable under standard storage conditions, with a shelf life of up to two years when stored at -20°C . Its stability may decrease at higher temperatures or in the presence of light. Long-term studies have shown that alismoxide maintains its anti-inflammatory and antibacterial properties over extended periods, although its potency may diminish with prolonged exposure to environmental factors .
Dosage Effects in Animal Models
The effects of alismoxide vary with different dosages in animal models. At low doses, alismoxide exhibits anti-inflammatory and antibacterial effects without significant toxicity . At higher doses, it may cause adverse effects, including hepatotoxicity and nephrotoxicity. Threshold effects have been observed, with a narrow therapeutic window between effective and toxic doses. Therefore, careful dosage optimization is crucial for maximizing the therapeutic benefits of alismoxide while minimizing potential risks .
Metabolic Pathways
Alismoxide is involved in several metabolic pathways, primarily related to its role as a sesquiterpenoid. It is metabolized by enzymes such as cytochrome P450, which catalyze its oxidation and subsequent conjugation with glucuronic acid . These metabolic transformations enhance the solubility and excretion of alismoxide. Additionally, alismoxide influences metabolic flux by modulating the activity of key enzymes involved in the urea cycle and nitric oxide synthesis .
Transport and Distribution
Within cells and tissues, alismoxide is transported and distributed through various mechanisms. It interacts with specific transporters and binding proteins that facilitate its uptake and localization . Alismoxide accumulates in target tissues, such as the liver and kidneys, where it exerts its therapeutic effects. Its distribution is influenced by factors such as tissue perfusion, binding affinity, and cellular uptake mechanisms .
Subcellular Localization
Alismoxide exhibits specific subcellular localization patterns that influence its activity and function. It is primarily localized in the cytoplasm and nucleus, where it interacts with key enzymes and transcription factors . Alismoxide’s localization is mediated by targeting signals and post-translational modifications that direct it to specific compartments or organelles. This subcellular distribution is crucial for its ability to modulate cellular processes and exert its therapeutic effects .
Vorbereitungsmethoden
Synthetic Routes and Reaction Conditions: Alismoxide can be synthesized through various chemical reactions. One common method involves the extraction of the compound from the rhizomes of Alisma orientale using organic solvents such as acetone. The extract is then subjected to chromatographic techniques to isolate alismoxide .
Industrial Production Methods: Industrial production of alismoxide typically involves large-scale extraction from plant sources. The process includes:
- Harvesting and drying the rhizomes of Alisma orientale.
- Grinding the dried rhizomes into a fine powder.
- Extracting the compound using solvents like acetone or ethanol.
- Purifying the extract through chromatography to obtain pure alismoxide .
Analyse Chemischer Reaktionen
Arten von Reaktionen: Alismoxid durchläuft verschiedene chemische Reaktionen, darunter:
Oxidation: this compound kann oxidiert werden, um verschiedene Derivate zu bilden, wie z. B. Alisol Q 23-Acetat.
Reduktion: Reduktionsreaktionen können die funktionellen Gruppen in this compound modifizieren, was zur Bildung von Verbindungen wie Alismol führt.
Substitution: This compound kann an Substitutionsreaktionen teilnehmen, bei denen funktionelle Gruppen durch andere Gruppen ersetzt werden
Häufige Reagenzien und Bedingungen:
Oxidation: Reagenzien wie Kaliumpermanganat oder Chromtrioxid werden üblicherweise verwendet.
Reduktion: Natriumborhydrid oder Lithiumaluminiumhydrid sind typische Reduktionsmittel.
Substitution: Verschiedene Halogenierungsmittel und Nukleophile können unter kontrollierten Bedingungen verwendet werden
Hauptprodukte:
Oxidation: Alisol Q 23-Acetat.
Reduktion: Alismol.
Substitution: Verschiedene halogenierte Derivate
Vergleich Mit ähnlichen Verbindungen
- Alisol B 23-acetate
- Alisol C 23-acetate
- Alismol
- 10-O-methyl-alismoxide
- 11-deoxyalisol C
Comparison: Alismoxide is unique due to its specific sesquiterpenoid structure, which imparts distinct biological activities. Compared to similar compounds like alisol B 23-acetate and alisol C 23-acetate, alismoxide exhibits stronger antibacterial and anti-inflammatory properties .
Eigenschaften
IUPAC Name |
(1S,3aR,4R,8aS)-1,4-dimethyl-7-propan-2-yl-2,3,3a,5,6,8a-hexahydroazulene-1,4-diol | |
---|---|---|
Source | PubChem | |
URL | https://pubchem.ncbi.nlm.nih.gov | |
Description | Data deposited in or computed by PubChem | |
InChI |
InChI=1S/C15H26O2/c1-10(2)11-5-7-14(3,16)12-6-8-15(4,17)13(12)9-11/h9-10,12-13,16-17H,5-8H2,1-4H3/t12-,13+,14-,15+/m1/s1 | |
Source | PubChem | |
URL | https://pubchem.ncbi.nlm.nih.gov | |
Description | Data deposited in or computed by PubChem | |
InChI Key |
IWQURBSTAIRNAE-BARDWOONSA-N | |
Source | PubChem | |
URL | https://pubchem.ncbi.nlm.nih.gov | |
Description | Data deposited in or computed by PubChem | |
Canonical SMILES |
CC(C)C1=CC2C(CCC2(C)O)C(CC1)(C)O | |
Source | PubChem | |
URL | https://pubchem.ncbi.nlm.nih.gov | |
Description | Data deposited in or computed by PubChem | |
Isomeric SMILES |
CC(C)C1=C[C@H]2[C@@H](CC[C@]2(C)O)[C@](CC1)(C)O | |
Source | PubChem | |
URL | https://pubchem.ncbi.nlm.nih.gov | |
Description | Data deposited in or computed by PubChem | |
Molecular Formula |
C15H26O2 | |
Source | PubChem | |
URL | https://pubchem.ncbi.nlm.nih.gov | |
Description | Data deposited in or computed by PubChem | |
Molecular Weight |
238.37 g/mol | |
Source | PubChem | |
URL | https://pubchem.ncbi.nlm.nih.gov | |
Description | Data deposited in or computed by PubChem | |
Retrosynthesis Analysis
AI-Powered Synthesis Planning: Our tool employs the Template_relevance Pistachio, Template_relevance Bkms_metabolic, Template_relevance Pistachio_ringbreaker, Template_relevance Reaxys, Template_relevance Reaxys_biocatalysis model, leveraging a vast database of chemical reactions to predict feasible synthetic routes.
One-Step Synthesis Focus: Specifically designed for one-step synthesis, it provides concise and direct routes for your target compounds, streamlining the synthesis process.
Accurate Predictions: Utilizing the extensive PISTACHIO, BKMS_METABOLIC, PISTACHIO_RINGBREAKER, REAXYS, REAXYS_BIOCATALYSIS database, our tool offers high-accuracy predictions, reflecting the latest in chemical research and data.
Strategy Settings
Precursor scoring | Relevance Heuristic |
---|---|
Min. plausibility | 0.01 |
Model | Template_relevance |
Template Set | Pistachio/Bkms_metabolic/Pistachio_ringbreaker/Reaxys/Reaxys_biocatalysis |
Top-N result to add to graph | 6 |
Feasible Synthetic Routes
Haftungsausschluss und Informationen zu In-Vitro-Forschungsprodukten
Bitte beachten Sie, dass alle Artikel und Produktinformationen, die auf BenchChem präsentiert werden, ausschließlich zu Informationszwecken bestimmt sind. Die auf BenchChem zum Kauf angebotenen Produkte sind speziell für In-vitro-Studien konzipiert, die außerhalb lebender Organismen durchgeführt werden. In-vitro-Studien, abgeleitet von dem lateinischen Begriff "in Glas", beinhalten Experimente, die in kontrollierten Laborumgebungen unter Verwendung von Zellen oder Geweben durchgeführt werden. Es ist wichtig zu beachten, dass diese Produkte nicht als Arzneimittel oder Medikamente eingestuft sind und keine Zulassung der FDA für die Vorbeugung, Behandlung oder Heilung von medizinischen Zuständen, Beschwerden oder Krankheiten erhalten haben. Wir müssen betonen, dass jede Form der körperlichen Einführung dieser Produkte in Menschen oder Tiere gesetzlich strikt untersagt ist. Es ist unerlässlich, sich an diese Richtlinien zu halten, um die Einhaltung rechtlicher und ethischer Standards in Forschung und Experiment zu gewährleisten.