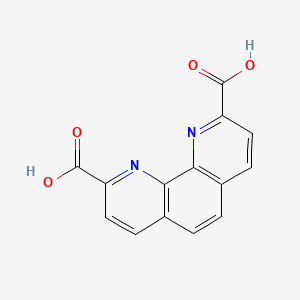
1,10-phenanthroline-2,9-dicarboxylic Acid
Übersicht
Beschreibung
1,10-Phenanthroline-2,9-dicarboxylic acid is an organic compound with the molecular formula C14H8N2O4. It is a derivative of phenanthroline, a heterocyclic aromatic compound, and is characterized by the presence of two carboxylic acid groups at the 2 and 9 positions of the phenanthroline ring. This compound is known for its versatility in various chemical reactions and its applications in scientific research.
Vorbereitungsmethoden
Synthetic Routes and Reaction Conditions: 1,10-Phenanthroline-2,9-dicarboxylic acid can be synthesized through several methods. One common approach involves the reaction of 1,10-phenanthroline with carbon dioxide under high pressure and temperature conditions. Another method includes the oxidation of 1,10-phenanthroline-2,9-dimethyl with potassium permanganate in an acidic medium .
Industrial Production Methods: Industrial production of this compound typically involves large-scale synthesis using optimized reaction conditions to ensure high yield and purity. The process may include steps such as recrystallization and purification to obtain the final product .
Analyse Chemischer Reaktionen
Types of Reactions: 1,10-Phenanthroline-2,9-dicarboxylic acid undergoes various chemical reactions, including:
Oxidation: The compound can be oxidized to form corresponding quinones.
Reduction: It can be reduced to form dihydro derivatives.
Substitution: The carboxylic acid groups can be substituted with other functional groups through esterification or amidation reactions.
Common Reagents and Conditions:
Oxidation: Potassium permanganate in acidic medium.
Reduction: Hydrogen gas in the presence of a palladium catalyst.
Substitution: Alcohols or amines in the presence of a dehydrating agent such as thionyl chloride.
Major Products Formed:
Oxidation: Quinones.
Reduction: Dihydro derivatives.
Substitution: Esters or amides.
Wissenschaftliche Forschungsanwendungen
Coordination Chemistry
1,10-Phenanthroline-2,9-dicarboxylic acid acts as a bidentate ligand, capable of forming stable complexes with transition metals. This property is exploited in several applications:
- Metal Ion Extraction : The compound has been used for the selective extraction of actinides and lanthanides from aqueous solutions. For example, studies have demonstrated its effectiveness in recovering uranium from seawater amidst competing vanadium species .
Metal Ion | Extraction Efficiency (%) | Reference |
---|---|---|
Uranium | Up to 40 | |
Europium | Higher than calixarene-type ligands |
Environmental Applications
The compound's ability to form complexes with metal ions makes it useful for environmental monitoring and remediation:
- Heavy Metal Detection : The complexation properties allow for the detection of heavy metals in water samples. Research has shown that the ligand can selectively bind to specific ions, facilitating their identification through spectroscopic methods .
Biochemical Applications
This compound has significant applications in biochemistry:
- Fluorescent Probes : Derivatives of this compound are used as markers in fluorescent immunoassays. For instance, the compound forms fluorescent complexes with europium ions, which are utilized for time-resolved fluorometric applications .
Application | Description | Reference |
---|---|---|
Fluorescent Immunoassay | Utilizes fluorescent complexes for protein labeling | |
Protein Labeling | Optimized conditions for labeling antibodies |
Synthesis of Derivatives
The synthesis of derivatives of this compound allows for tailored applications:
- Functionalization : Various functional groups can be introduced to modify the properties of the base compound. Research has explored methods to synthesize derivatives that enhance solubility and reactivity in specific environments .
Case Study 1: Selective Recovery of Uranium
A study investigated the use of this compound as a ligand for the selective recovery of uranium from seawater. The ligand demonstrated a high affinity for uranium ions while effectively competing against vanadium species. The extraction efficiency was quantified using UV-visible spectroscopy to analyze complex formation .
Case Study 2: Fluorescent Labeling Techniques
In another study focusing on fluorescent immunoassays, the compound was utilized to label proteins effectively. The resulting fluorescent complexes exhibited long lifetimes suitable for time-resolved measurements. Parameters such as pH and solvent type were optimized to enhance labeling efficiency without compromising biological activity .
Wirkmechanismus
The mechanism of action of 1,10-phenanthroline-2,9-dicarboxylic acid involves its ability to chelate metal ions. The nitrogen atoms in the phenanthroline ring and the carboxylic acid groups coordinate with metal ions, forming stable complexes. These complexes can interact with biological molecules, inhibiting enzyme activity or altering cellular processes .
Vergleich Mit ähnlichen Verbindungen
1,10-Phenanthroline: Lacks the carboxylic acid groups, making it less versatile in certain reactions.
2,2’-Bipyridine: Another chelating agent with similar properties but different coordination chemistry.
Ethylenediaminetetraacetic acid (EDTA): A widely used chelating agent with multiple carboxylic acid groups.
Uniqueness: 1,10-Phenanthroline-2,9-dicarboxylic acid is unique due to its dual functionality as both a chelating agent and a reactive intermediate. The presence of carboxylic acid groups enhances its reactivity and allows for the formation of a wide range of derivatives .
Biologische Aktivität
1,10-Phenanthroline-2,9-dicarboxylic acid (PDCA) is a compound of significant interest in various fields of biological and chemical research. Its unique structure allows for a range of biological activities, particularly in chelation chemistry and fluorescent applications. This article reviews the biological activity of PDCA, highlighting its mechanisms, applications, and relevant research findings.
Chemical Structure and Properties
PDCA is characterized by its phenanthroline backbone with two carboxylic acid groups at the 2 and 9 positions. This structure enables the formation of stable complexes with metal ions, particularly lanthanides, which are crucial for various biochemical applications.
Chelation Properties
PDCA exhibits strong chelating properties, particularly with lanthanide ions such as europium (Eu³⁺) and neodymium (Nd³⁺). The ability to form stable chelates is important for its use in fluorescent assays and as a marker in immunoassays.
- Fluorescent Properties : The chelates formed between PDCA and lanthanides exhibit high fluorescence quantum yields. This property is utilized in various analytical techniques, enhancing the sensitivity of detection methods in biochemical assays .
Applications in Immunoassays
PDCA derivatives have been developed for use as fluorescent markers in immunoassays. These derivatives can be covalently linked to proteins, allowing for the detection of specific antigens through fluorescence. The use of PDCA in this context improves the sensitivity and specificity of immunoassays due to its strong luminescent properties .
Complexation Studies
Research has demonstrated that PDCA exhibits selective complexation behavior with actinides such as americium (Am³⁺) and neodymium (Nd³⁺). Spectrophotometric studies reveal that PDCA forms stable complexes with these ions, indicating potential applications in nuclear chemistry and environmental monitoring .
Case Studies
- Fluorescent Probes : A study highlighted the synthesis of a new fluorescent probe based on PDCA, which was optimized for protein labeling. This probe demonstrated enhanced fluorescence properties compared to traditional markers .
- Selectivity Towards Metal Ions : Research indicated that PDCA shows a high selectivity for UO₂²⁺ over other competing metal ions like VO₂⁺. This selectivity is crucial for applications in radiochemistry and environmental analysis .
- Crystal Structure Analysis : The crystal structure of PDCA has been investigated, revealing insights into its self-association properties and proton transfer mechanisms. Such structural studies are essential for understanding the compound's reactivity and interaction with biological systems .
Data Tables
Property | Value |
---|---|
Molecular Formula | C₁₂H₈N₂O₄ |
Melting Point | 194-198 °C |
Solubility | Soluble in water |
Chelation Strength | High (with lanthanides) |
Q & A
Basic Research Questions
Q. What are the key synthetic routes for 1,10-phenanthroline-2,9-dicarboxylic acid (PDA), and how do they influence purity and yield?
PDA is synthesized via oxidation of 2,9-dimethyl-1,10-phenanthroline. A two-step method involves:
- Step 1 : Oxidation with SeO₂ in dioxane under reflux to yield 1,10-phenanthroline-2,9-dicarbaldehyde .
- Step 2 : Further oxidation with 70% HNO₃ to convert the aldehyde groups to carboxylic acids, followed by recrystallization from methanol . Yield and purity depend on reaction time, stoichiometry of SeO₂, and nitric acid concentration. Impurities (e.g., unreacted aldehyde) can be monitored via FT-IR or HPLC.
Q. How does the crystal structure of PDA influence its coordination chemistry?
PDA crystallizes in a monoclinic system (space group P 1 21/a 1) with unit cell dimensions a = 6.9434 Å, b = 23.8950 Å, and c = 10.1897 Å . The planar geometry of PDA, with carboxylate groups at positions 2 and 9, preorganizes the ligand for metal binding, reducing entropic penalties during complexation. This rigidity enhances thermodynamic stability in metal complexes, as seen in Th(IV) and U(VI) complexes .
Q. What spectroscopic methods are critical for characterizing PDA and its metal complexes?
- FT-IR : Identifies carboxylate stretching vibrations (~1700 cm⁻¹ for free COOH, ~1600–1400 cm⁻¹ for deprotonated COO⁻) and pyridyl N coordination shifts .
- UV-Vis : Monitors ligand-to-metal charge transfer (LMCT) bands in complexes (e.g., uranyl-PDA complexes show distinct absorption at ~300–400 nm) .
- X-ray crystallography : Resolves bond lengths (e.g., U–O = 2.351 Å, U–N = 2.558 Å in [UO₂(PDA)]) and confirms planarity .
Advanced Research Questions
Q. How can PDA be rationally designed into functional materials for sensing applications?
PDA’s preorganized structure enables integration into Fe-N-C nanosheets for electrochemiluminescence (ECL) sensors. Example:
- Fe-N-C/PDA synthesis : PDA acts as a ligand precursor, coordinating Fe³⁺ to form highly dispersed Fe-N-C catalysts.
- ECL mechanism : The nanosheet enhances tetracycline detection via in situ generation of reactive oxygen species (ROS), achieving a detection limit of 0.03 nM . Key parameters include Fe/PDA molar ratio, pyrolysis temperature, and electrolyte pH.
Q. What explains PDA’s exceptional selectivity for actinides over lanthanides in solvent extraction?
PDA’s diamide derivatives (e.g., N,N,N’,N’-tetraoctyl-PDA) exhibit size- and charge-based selectivity:
- Thermodynamic stability : Log K₁ for Th(IV)-PDA is 25.7, driven by synergistic pyridyl-carboxylate binding and minimized steric strain .
- Solvent effects : In HNO₃ media, PDA diamides preferentially extract Am(III) over Eu(III) (separation factor SFAm/Eu > 40) due to stronger orbital overlap with 5f vs. 4f electrons . Optimization involves tuning alkyl substituents on the amide groups to balance lipophilicity and steric hindrance .
Q. How do crystallographic data resolve contradictions in PDA-metal complex stability across different studies?
Discrepancies in stability constants (e.g., Th(IV) vs. U(VI) complexes) arise from ligand distortion in crowded coordination spheres. For example:
- Planar vs. bowed ligands : In [Th(PDA)₂(H₂O)₂], PDA is bowed (Th out-of-plane), increasing strain and reducing log K₁ compared to planar [UO₂(PDA)] .
- DFT validation : Computational studies show PDA’s reorganization energy is lower in uranyl complexes, aligning with experimental stability trends .
Q. What strategies mitigate challenges in synthesizing PDA-based coordination polymers?
- Hydrothermal synthesis : High-temperature aqueous conditions (e.g., 120°C, 24 hr) promote network formation, as seen in [Ni(L)(H₂O)₃]·H₂O, which forms a 3D H-bonded framework .
- pH control : Deprotonation of carboxylates at pH > 4 enhances metal binding, critical for avoiding precipitate formation in Th(IV) complexes .
Q. Methodological Considerations
Q. How should researchers analyze conflicting data on PDA’s metal ion size selectivity?
- Ionic radius vs. charge : PDA favors large, high-charge ions (e.g., Th⁴⁺, ionic radius ~1.0 Å) over smaller ions (e.g., Zn²⁺, 0.74 Å). Cross-validate using UV-Vis titration (e.g., competition experiments with EDTA) .
- Solvent screening : Test extraction efficiency in varying HNO₃ concentrations (0.1–3 M) and diluents (e.g., n-dodecane vs. ionic liquids) to isolate size/charge effects .
Q. What are best practices for optimizing PDA-based sensors for environmental contaminants?
Eigenschaften
IUPAC Name |
1,10-phenanthroline-2,9-dicarboxylic acid | |
---|---|---|
Source | PubChem | |
URL | https://pubchem.ncbi.nlm.nih.gov | |
Description | Data deposited in or computed by PubChem | |
InChI |
InChI=1S/C14H8N2O4/c17-13(18)9-5-3-7-1-2-8-4-6-10(14(19)20)16-12(8)11(7)15-9/h1-6H,(H,17,18)(H,19,20) | |
Source | PubChem | |
URL | https://pubchem.ncbi.nlm.nih.gov | |
Description | Data deposited in or computed by PubChem | |
InChI Key |
FXSVCROWUPWXBP-UHFFFAOYSA-N | |
Source | PubChem | |
URL | https://pubchem.ncbi.nlm.nih.gov | |
Description | Data deposited in or computed by PubChem | |
Canonical SMILES |
C1=CC2=C(C3=C1C=CC(=N3)C(=O)O)N=C(C=C2)C(=O)O | |
Source | PubChem | |
URL | https://pubchem.ncbi.nlm.nih.gov | |
Description | Data deposited in or computed by PubChem | |
Molecular Formula |
C14H8N2O4 | |
Source | PubChem | |
URL | https://pubchem.ncbi.nlm.nih.gov | |
Description | Data deposited in or computed by PubChem | |
DSSTOX Substance ID |
DTXSID70428797 | |
Record name | 1,10-phenanthroline-2,9-dicarboxylic Acid | |
Source | EPA DSSTox | |
URL | https://comptox.epa.gov/dashboard/DTXSID70428797 | |
Description | DSSTox provides a high quality public chemistry resource for supporting improved predictive toxicology. | |
Molecular Weight |
268.22 g/mol | |
Source | PubChem | |
URL | https://pubchem.ncbi.nlm.nih.gov | |
Description | Data deposited in or computed by PubChem | |
CAS No. |
57709-61-2 | |
Record name | 1,10-phenanthroline-2,9-dicarboxylic Acid | |
Source | EPA DSSTox | |
URL | https://comptox.epa.gov/dashboard/DTXSID70428797 | |
Description | DSSTox provides a high quality public chemistry resource for supporting improved predictive toxicology. | |
Retrosynthesis Analysis
AI-Powered Synthesis Planning: Our tool employs the Template_relevance Pistachio, Template_relevance Bkms_metabolic, Template_relevance Pistachio_ringbreaker, Template_relevance Reaxys, Template_relevance Reaxys_biocatalysis model, leveraging a vast database of chemical reactions to predict feasible synthetic routes.
One-Step Synthesis Focus: Specifically designed for one-step synthesis, it provides concise and direct routes for your target compounds, streamlining the synthesis process.
Accurate Predictions: Utilizing the extensive PISTACHIO, BKMS_METABOLIC, PISTACHIO_RINGBREAKER, REAXYS, REAXYS_BIOCATALYSIS database, our tool offers high-accuracy predictions, reflecting the latest in chemical research and data.
Strategy Settings
Precursor scoring | Relevance Heuristic |
---|---|
Min. plausibility | 0.01 |
Model | Template_relevance |
Template Set | Pistachio/Bkms_metabolic/Pistachio_ringbreaker/Reaxys/Reaxys_biocatalysis |
Top-N result to add to graph | 6 |
Feasible Synthetic Routes
Haftungsausschluss und Informationen zu In-Vitro-Forschungsprodukten
Bitte beachten Sie, dass alle Artikel und Produktinformationen, die auf BenchChem präsentiert werden, ausschließlich zu Informationszwecken bestimmt sind. Die auf BenchChem zum Kauf angebotenen Produkte sind speziell für In-vitro-Studien konzipiert, die außerhalb lebender Organismen durchgeführt werden. In-vitro-Studien, abgeleitet von dem lateinischen Begriff "in Glas", beinhalten Experimente, die in kontrollierten Laborumgebungen unter Verwendung von Zellen oder Geweben durchgeführt werden. Es ist wichtig zu beachten, dass diese Produkte nicht als Arzneimittel oder Medikamente eingestuft sind und keine Zulassung der FDA für die Vorbeugung, Behandlung oder Heilung von medizinischen Zuständen, Beschwerden oder Krankheiten erhalten haben. Wir müssen betonen, dass jede Form der körperlichen Einführung dieser Produkte in Menschen oder Tiere gesetzlich strikt untersagt ist. Es ist unerlässlich, sich an diese Richtlinien zu halten, um die Einhaltung rechtlicher und ethischer Standards in Forschung und Experiment zu gewährleisten.