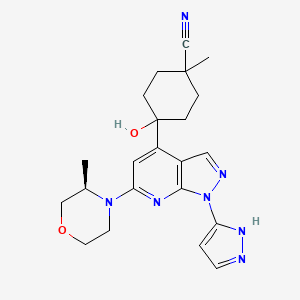
Atr-IN-9
- Klicken Sie auf QUICK INQUIRY, um ein Angebot von unserem Expertenteam zu erhalten.
- Mit qualitativ hochwertigen Produkten zu einem WETTBEWERBSFÄHIGEN Preis können Sie sich mehr auf Ihre Forschung konzentrieren.
Übersicht
Beschreibung
Atr-IN-9 is a potent inhibitor of the Ataxia-telangiectasia and RAD-3-related protein kinase (ATR). This compound has shown significant efficacy in inhibiting ATR with an IC50 value of 10 nanomolar . ATR is a crucial protein kinase involved in the DNA damage response, particularly in the repair of DNA double-strand breaks and the stabilization of replication forks.
Vorbereitungsmethoden
The synthesis of Atr-IN-9 involves several steps, starting with the preparation of the core structure, followed by the introduction of various functional groups. The synthetic route typically includes:
Formation of the Core Structure: This involves the construction of the central scaffold of the molecule through a series of condensation and cyclization reactions.
Functional Group Introduction: Various functional groups are introduced to the core structure through substitution reactions, often using reagents such as halides and organometallic compounds.
Purification and Characterization: The final product is purified using techniques such as column chromatography and characterized using spectroscopic methods like nuclear magnetic resonance (NMR) and mass spectrometry (MS).
Industrial production methods for this compound would likely involve scaling up these synthetic routes, optimizing reaction conditions to increase yield and purity, and ensuring compliance with regulatory standards for pharmaceutical production.
Analyse Chemischer Reaktionen
Atr-IN-9 undergoes several types of chemical reactions, including:
Oxidation: This reaction involves the addition of oxygen or the removal of hydrogen, often using oxidizing agents such as hydrogen peroxide or potassium permanganate.
Reduction: This involves the addition of hydrogen or the removal of oxygen, typically using reducing agents like sodium borohydride or lithium aluminum hydride.
Substitution: This reaction involves the replacement of one functional group with another, often using reagents such as halides or organometallic compounds.
Common reagents and conditions used in these reactions include organic solvents like dichloromethane or tetrahydrofuran, catalysts such as palladium on carbon, and specific temperature and pressure conditions to optimize reaction rates and yields. The major products formed from these reactions depend on the specific functional groups involved and the reaction conditions used.
Wissenschaftliche Forschungsanwendungen
Atr-IN-9 has a wide range of scientific research applications, including:
Chemistry: It is used as a tool compound to study the role of ATR in DNA damage response and repair mechanisms.
Biology: Researchers use this compound to investigate the cellular pathways regulated by ATR and to understand how ATR inhibition affects cell cycle progression and apoptosis.
Medicine: this compound is being explored as a potential therapeutic agent for cancer treatment, particularly in tumors with defects in DNA repair pathways.
Industry: The compound is used in the development of new pharmaceuticals and as a reference standard in quality control processes.
Wirkmechanismus
Atr-IN-9 exerts its effects by inhibiting the activity of ATR, a serine/threonine protein kinase that plays a critical role in the DNA damage response. ATR is activated in response to DNA damage and replication stress, leading to the phosphorylation of downstream targets such as checkpoint kinase 1 (CHK1). This activation results in cell cycle arrest, allowing time for DNA repair. By inhibiting ATR, this compound prevents the activation of these downstream targets, leading to increased DNA damage and cell death, particularly in cancer cells with defective DNA repair mechanisms .
Vergleich Mit ähnlichen Verbindungen
Atr-IN-9 is unique among ATR inhibitors due to its high potency and selectivity. Similar compounds include:
AZD6738 (Ceralasertib): Another potent ATR inhibitor with similar applications in cancer research
VE-821: An ATR inhibitor used in preclinical studies to investigate the role of ATR in DNA damage response.
ETP-46464: A selective ATR inhibitor with applications in studying ATR-mediated signaling pathways.
Compared to these compounds, this compound has shown superior potency and selectivity, making it a valuable tool in both basic research and therapeutic development.
Eigenschaften
Molekularformel |
C22H27N7O2 |
---|---|
Molekulargewicht |
421.5 g/mol |
IUPAC-Name |
4-hydroxy-1-methyl-4-[6-[(3R)-3-methylmorpholin-4-yl]-1-(1H-pyrazol-5-yl)pyrazolo[3,4-b]pyridin-4-yl]cyclohexane-1-carbonitrile |
InChI |
InChI=1S/C22H27N7O2/c1-15-13-31-10-9-28(15)19-11-17(22(30)6-4-21(2,14-23)5-7-22)16-12-25-29(20(16)26-19)18-3-8-24-27-18/h3,8,11-12,15,30H,4-7,9-10,13H2,1-2H3,(H,24,27)/t15-,21?,22?/m1/s1 |
InChI-Schlüssel |
WBVUJJIWUYNDHF-IYJORBIASA-N |
Isomerische SMILES |
C[C@@H]1COCCN1C2=NC3=C(C=NN3C4=CC=NN4)C(=C2)C5(CCC(CC5)(C)C#N)O |
Kanonische SMILES |
CC1COCCN1C2=NC3=C(C=NN3C4=CC=NN4)C(=C2)C5(CCC(CC5)(C)C#N)O |
Herkunft des Produkts |
United States |
Haftungsausschluss und Informationen zu In-Vitro-Forschungsprodukten
Bitte beachten Sie, dass alle Artikel und Produktinformationen, die auf BenchChem präsentiert werden, ausschließlich zu Informationszwecken bestimmt sind. Die auf BenchChem zum Kauf angebotenen Produkte sind speziell für In-vitro-Studien konzipiert, die außerhalb lebender Organismen durchgeführt werden. In-vitro-Studien, abgeleitet von dem lateinischen Begriff "in Glas", beinhalten Experimente, die in kontrollierten Laborumgebungen unter Verwendung von Zellen oder Geweben durchgeführt werden. Es ist wichtig zu beachten, dass diese Produkte nicht als Arzneimittel oder Medikamente eingestuft sind und keine Zulassung der FDA für die Vorbeugung, Behandlung oder Heilung von medizinischen Zuständen, Beschwerden oder Krankheiten erhalten haben. Wir müssen betonen, dass jede Form der körperlichen Einführung dieser Produkte in Menschen oder Tiere gesetzlich strikt untersagt ist. Es ist unerlässlich, sich an diese Richtlinien zu halten, um die Einhaltung rechtlicher und ethischer Standards in Forschung und Experiment zu gewährleisten.