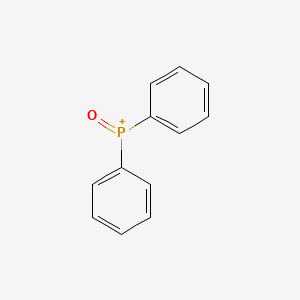
Diphenylphosphine oxide
Übersicht
Beschreibung
Diphenylphosphine oxide is an organophosphorus compound with the chemical formula (C₆H₅)₂P(O)H. It is a white solid that is soluble in polar organic solvents. This compound is notable for its use in various chemical reactions and applications, particularly in organic synthesis and as a ligand in coordination chemistry .
Wissenschaftliche Forschungsanwendungen
Diphenylphosphine oxide has a wide range of applications in scientific research:
Chemistry: It is used as a ligand in coordination chemistry and in the synthesis of various organophosphorus compounds.
Biology: It serves as a precursor for biologically active molecules.
Medicine: It is involved in the synthesis of pharmaceuticals and other bioactive compounds.
Industry: It is used in the production of flame retardants for epoxy resins and other materials .
Wirkmechanismus
Safety and Hazards
Zukünftige Richtungen
Biochemische Analyse
Biochemical Properties
Diphenylphosphine oxide plays a significant role in biochemical reactions, particularly in the synthesis of various organophosphorus compounds. It interacts with enzymes and proteins involved in these reactions. For instance, it is used as a ligand in catalytic reactions, where it binds to transition metals to form complexes that facilitate the reaction. The nature of these interactions is typically through coordination bonds between the phosphorus atom of this compound and the metal center of the enzyme or protein .
Cellular Effects
This compound influences various cellular processes. It has been observed to affect cell signaling pathways, gene expression, and cellular metabolism. For example, in the presence of this compound, certain signaling pathways may be activated or inhibited, leading to changes in gene expression. This compound can also impact cellular metabolism by interacting with metabolic enzymes, thereby altering the metabolic flux within the cell .
Molecular Mechanism
The molecular mechanism of this compound involves its binding interactions with biomolecules. It can act as an enzyme inhibitor or activator, depending on the specific enzyme it interacts with. For instance, this compound can inhibit the activity of certain enzymes by binding to their active sites, preventing substrate binding and subsequent catalysis. Additionally, it can induce changes in gene expression by interacting with transcription factors or other regulatory proteins .
Temporal Effects in Laboratory Settings
In laboratory settings, the effects of this compound can change over time. This compound is relatively stable under standard conditions, but it can degrade under certain conditions, such as exposure to strong acids or bases. Long-term studies have shown that this compound can have lasting effects on cellular function, including sustained changes in gene expression and metabolic activity .
Dosage Effects in Animal Models
The effects of this compound vary with different dosages in animal models. At low doses, it may have minimal impact on cellular function, while at higher doses, it can cause significant changes, including toxic or adverse effects. For example, high doses of this compound have been associated with oxidative stress and cellular damage in animal studies .
Metabolic Pathways
This compound is involved in several metabolic pathways. It interacts with enzymes such as phosphatases and kinases, which play crucial roles in cellular metabolism. These interactions can affect the levels of various metabolites and alter the metabolic flux within the cell. For instance, this compound can inhibit the activity of certain phosphatases, leading to an accumulation of phosphorylated metabolites .
Transport and Distribution
Within cells and tissues, this compound is transported and distributed through various mechanisms. It can interact with transporters and binding proteins that facilitate its movement across cellular membranes. Additionally, this compound can accumulate in specific cellular compartments, depending on its interactions with intracellular proteins and organelles .
Subcellular Localization
The subcellular localization of this compound is influenced by its chemical properties and interactions with cellular components. It can be directed to specific compartments or organelles through targeting signals or post-translational modifications. For example, this compound may localize to the mitochondria, where it can affect mitochondrial function and energy metabolism .
Vorbereitungsmethoden
Synthetic Routes and Reaction Conditions: Diphenylphosphine oxide can be synthesized through several methods:
- One common method involves the reaction of diethylphosphite with phenylmagnesium bromide, followed by acid workup. The reaction proceeds as follows:
Reaction of Phosphonic Esters with Grignard Reagents: (C2H5O)2P(O)H+3C6H5MgBr→(C6H5)2P(O)MgBr+C2H5OMgBr
(C6H5)2P(O)MgBr+HCl→(C6H5)2P(O)H+MgBrCl
Partial Hydrolysis of Chlorodiphenylphosphine: Another method involves the partial hydrolysis of chlorodiphenylphosphine or diphenylphosphine.
Industrial Production Methods: Industrial production methods for this compound typically involve large-scale synthesis using the aforementioned reactions, optimized for yield and purity. The choice of method depends on the availability of starting materials and the desired scale of production.
Analyse Chemischer Reaktionen
Types of Reactions: Diphenylphosphine oxide undergoes various chemical reactions, including:
Oxidation: It can be oxidized to form diphenylphosphinic acid.
Reduction: It can be reduced to diphenylphosphine using reducing agents like lithium aluminum hydride.
Common Reagents and Conditions:
Oxidation: Common oxidizing agents include hydrogen peroxide and other peroxides.
Reduction: Lithium aluminum hydride is a typical reducing agent.
Substitution: Palladium catalysts are often used in Buchwald-Hartwig coupling reactions.
Major Products Formed:
Oxidation: Diphenylphosphinic acid.
Reduction: Diphenylphosphine.
Substitution: Various organophosphorus compounds with diphenylphosphino substituents.
Vergleich Mit ähnlichen Verbindungen
Chlorodiphenylphosphine: Similar in structure but contains a chlorine atom instead of an oxygen atom.
Diphenylphosphine: The reduced form of diphenylphosphine oxide.
Triphenylphosphine oxide: Contains an additional phenyl group compared to this compound.
Uniqueness: this compound is unique due to its balance of reactivity and stability, making it a versatile reagent in organic synthesis. Its ability to act as both an oxidizing and reducing agent, as well as its role as a ligand, sets it apart from other similar compounds .
Eigenschaften
IUPAC Name |
oxo(diphenyl)phosphanium | |
---|---|---|
Source | PubChem | |
URL | https://pubchem.ncbi.nlm.nih.gov | |
Description | Data deposited in or computed by PubChem | |
InChI |
InChI=1S/C12H10OP/c13-14(11-7-3-1-4-8-11)12-9-5-2-6-10-12/h1-10H/q+1 | |
Source | PubChem | |
URL | https://pubchem.ncbi.nlm.nih.gov | |
Description | Data deposited in or computed by PubChem | |
InChI Key |
YFPJFKYCVYXDJK-UHFFFAOYSA-N | |
Source | PubChem | |
URL | https://pubchem.ncbi.nlm.nih.gov | |
Description | Data deposited in or computed by PubChem | |
Canonical SMILES |
C1=CC=C(C=C1)[P+](=O)C2=CC=CC=C2 | |
Source | PubChem | |
URL | https://pubchem.ncbi.nlm.nih.gov | |
Description | Data deposited in or computed by PubChem | |
Molecular Formula |
C12H10OP+ | |
Source | PubChem | |
URL | https://pubchem.ncbi.nlm.nih.gov | |
Description | Data deposited in or computed by PubChem | |
DSSTOX Substance ID |
DTXSID60422562 | |
Record name | Diphenylphosphine oxide | |
Source | EPA DSSTox | |
URL | https://comptox.epa.gov/dashboard/DTXSID60422562 | |
Description | DSSTox provides a high quality public chemistry resource for supporting improved predictive toxicology. | |
Molecular Weight |
201.18 g/mol | |
Source | PubChem | |
URL | https://pubchem.ncbi.nlm.nih.gov | |
Description | Data deposited in or computed by PubChem | |
CAS No. |
4559-70-0 | |
Record name | Diphenylphosphine oxide | |
Source | ChemIDplus | |
URL | https://pubchem.ncbi.nlm.nih.gov/substance/?source=chemidplus&sourceid=0004559700 | |
Description | ChemIDplus is a free, web search system that provides access to the structure and nomenclature authority files used for the identification of chemical substances cited in National Library of Medicine (NLM) databases, including the TOXNET system. | |
Record name | Diphenylphosphine oxide | |
Source | DTP/NCI | |
URL | https://dtp.cancer.gov/dtpstandard/servlet/dwindex?searchtype=NSC&outputformat=html&searchlist=77604 | |
Description | The NCI Development Therapeutics Program (DTP) provides services and resources to the academic and private-sector research communities worldwide to facilitate the discovery and development of new cancer therapeutic agents. | |
Explanation | Unless otherwise indicated, all text within NCI products is free of copyright and may be reused without our permission. Credit the National Cancer Institute as the source. | |
Record name | Diphenylphosphine oxide | |
Source | EPA DSSTox | |
URL | https://comptox.epa.gov/dashboard/DTXSID60422562 | |
Description | DSSTox provides a high quality public chemistry resource for supporting improved predictive toxicology. | |
Record name | (phenylphosphoroso)benzene | |
Source | European Chemicals Agency (ECHA) | |
URL | https://echa.europa.eu/information-on-chemicals | |
Description | The European Chemicals Agency (ECHA) is an agency of the European Union which is the driving force among regulatory authorities in implementing the EU's groundbreaking chemicals legislation for the benefit of human health and the environment as well as for innovation and competitiveness. | |
Explanation | Use of the information, documents and data from the ECHA website is subject to the terms and conditions of this Legal Notice, and subject to other binding limitations provided for under applicable law, the information, documents and data made available on the ECHA website may be reproduced, distributed and/or used, totally or in part, for non-commercial purposes provided that ECHA is acknowledged as the source: "Source: European Chemicals Agency, http://echa.europa.eu/". Such acknowledgement must be included in each copy of the material. ECHA permits and encourages organisations and individuals to create links to the ECHA website under the following cumulative conditions: Links can only be made to webpages that provide a link to the Legal Notice page. | |
Record name | DIPHENYLPHOSPHINE OXIDE | |
Source | FDA Global Substance Registration System (GSRS) | |
URL | https://gsrs.ncats.nih.gov/ginas/app/beta/substances/XXW49HG6X3 | |
Description | The FDA Global Substance Registration System (GSRS) enables the efficient and accurate exchange of information on what substances are in regulated products. Instead of relying on names, which vary across regulatory domains, countries, and regions, the GSRS knowledge base makes it possible for substances to be defined by standardized, scientific descriptions. | |
Explanation | Unless otherwise noted, the contents of the FDA website (www.fda.gov), both text and graphics, are not copyrighted. They are in the public domain and may be republished, reprinted and otherwise used freely by anyone without the need to obtain permission from FDA. Credit to the U.S. Food and Drug Administration as the source is appreciated but not required. | |
Retrosynthesis Analysis
AI-Powered Synthesis Planning: Our tool employs the Template_relevance Pistachio, Template_relevance Bkms_metabolic, Template_relevance Pistachio_ringbreaker, Template_relevance Reaxys, Template_relevance Reaxys_biocatalysis model, leveraging a vast database of chemical reactions to predict feasible synthetic routes.
One-Step Synthesis Focus: Specifically designed for one-step synthesis, it provides concise and direct routes for your target compounds, streamlining the synthesis process.
Accurate Predictions: Utilizing the extensive PISTACHIO, BKMS_METABOLIC, PISTACHIO_RINGBREAKER, REAXYS, REAXYS_BIOCATALYSIS database, our tool offers high-accuracy predictions, reflecting the latest in chemical research and data.
Strategy Settings
Precursor scoring | Relevance Heuristic |
---|---|
Min. plausibility | 0.01 |
Model | Template_relevance |
Template Set | Pistachio/Bkms_metabolic/Pistachio_ringbreaker/Reaxys/Reaxys_biocatalysis |
Top-N result to add to graph | 6 |
Feasible Synthetic Routes
Haftungsausschluss und Informationen zu In-Vitro-Forschungsprodukten
Bitte beachten Sie, dass alle Artikel und Produktinformationen, die auf BenchChem präsentiert werden, ausschließlich zu Informationszwecken bestimmt sind. Die auf BenchChem zum Kauf angebotenen Produkte sind speziell für In-vitro-Studien konzipiert, die außerhalb lebender Organismen durchgeführt werden. In-vitro-Studien, abgeleitet von dem lateinischen Begriff "in Glas", beinhalten Experimente, die in kontrollierten Laborumgebungen unter Verwendung von Zellen oder Geweben durchgeführt werden. Es ist wichtig zu beachten, dass diese Produkte nicht als Arzneimittel oder Medikamente eingestuft sind und keine Zulassung der FDA für die Vorbeugung, Behandlung oder Heilung von medizinischen Zuständen, Beschwerden oder Krankheiten erhalten haben. Wir müssen betonen, dass jede Form der körperlichen Einführung dieser Produkte in Menschen oder Tiere gesetzlich strikt untersagt ist. Es ist unerlässlich, sich an diese Richtlinien zu halten, um die Einhaltung rechtlicher und ethischer Standards in Forschung und Experiment zu gewährleisten.