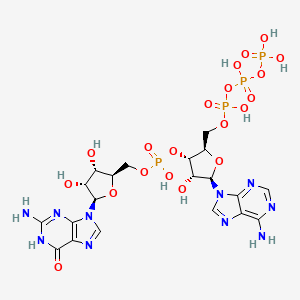
pppApG
- Klicken Sie auf QUICK INQUIRY, um ein Angebot von unserem Expertenteam zu erhalten.
- Mit qualitativ hochwertigen Produkten zu einem WETTBEWERBSFÄHIGEN Preis können Sie sich mehr auf Ihre Forschung konzentrieren.
Übersicht
Beschreibung
The compound 5’-Triphosphoadenylyl-(3’→5’)-guanosine, commonly referred to as pppApG, is a linear dinucleotide. It consists of a 5’-adenosine triphosphate unit connected to a 5’-guanosine monophosphate unit via a 3’→5’ linkage . This compound is a potential endogenous metabolite of the metazoan second messenger 2’,3’-cyclic guanosine monophosphate-adenosine monophosphate and/or the bacterial signaling nucleotide 3’,3’-cyclic guanosine monophosphate-adenosine monophosphate .
Vorbereitungsmethoden
Synthetic Routes and Reaction Conditions
The synthesis of 5’-Triphosphoadenylyl-(3’→5’)-guanosine can be achieved through in vitro transcription using DNA-dependent bacteriophage T7 RNA polymerase . The DNA template is prepared by annealing specific oligonucleotides, and the transcription reaction contains adenosine triphosphate and guanosine triphosphate . This method allows for the preparation of short oligoribonucleotides with different phosphorylation states and modifications on the 5’ terminus .
Industrial Production Methods
Industrial production of 5’-Triphosphoadenylyl-(3’→5’)-guanosine typically involves large-scale in vitro transcription processes. The compound is stored as an aqueous solution at -20°C to maintain stability . The product is shipped on gel packs and has a shelf life of 12 months after delivery .
Analyse Chemischer Reaktionen
Types of Reactions
5’-Triphosphoadenylyl-(3’→5’)-guanosine undergoes various biochemical reactions, including:
Phosphorylation: The addition of phosphate groups to the molecule.
Hydrolysis: The cleavage of chemical bonds by the addition of water.
Cyclization: The formation of cyclic structures from linear molecules.
Common Reagents and Conditions
Common reagents used in these reactions include adenosine triphosphate, guanosine triphosphate, and specific enzymes such as RNA polymerases . The reactions typically occur under physiological conditions, with optimal pH and temperature maintained to ensure enzyme activity .
Major Products
The major products formed from these reactions include cyclic guanosine monophosphate-adenosine monophosphate and other cyclic dinucleotides .
Wissenschaftliche Forschungsanwendungen
5’-Triphosphoadenylyl-(3’→5’)-guanosine has several scientific research applications:
Chemistry: Used in the study of nucleotide interactions and enzyme mechanisms.
Medicine: Investigated for its potential role in antiviral therapies and immune response modulation.
Industry: Utilized in the production of synthetic RNA molecules for various applications.
Wirkmechanismus
The mechanism of action of 5’-Triphosphoadenylyl-(3’→5’)-guanosine involves its role as a substrate in the synthesis of viral RNA and complementary RNA . The compound is used in primer-independent initiation of RNA synthesis, where it binds to specific positions on the RNA template and facilitates elongation . This process is crucial for the replication and transcription of viral genomes.
Vergleich Mit ähnlichen Verbindungen
Similar Compounds
- 2’,3’-cyclic guanosine monophosphate-adenosine monophosphate
- 3’,3’-cyclic guanosine monophosphate-adenosine monophosphate
- 5’-Triphosphoadenylyl-(3’→5’)-cytidine
Uniqueness
5’-Triphosphoadenylyl-(3’→5’)-guanosine is unique due to its specific linear dinucleotide structure and its role as an intermediate in the synthesis of cyclic dinucleotides . Unlike its cyclic counterparts, it serves as a precursor in the enzymatic production of cyclic guanosine monophosphate-adenosine monophosphate, highlighting its importance in biochemical pathways .
Eigenschaften
Molekularformel |
C20H28N10O20P4 |
---|---|
Molekulargewicht |
852.4 g/mol |
IUPAC-Name |
[[(2R,3S,4R,5R)-3-[[(2R,3S,4R,5R)-5-(2-amino-6-oxo-1H-purin-9-yl)-3,4-dihydroxyoxolan-2-yl]methoxy-hydroxyphosphoryl]oxy-5-(6-aminopurin-9-yl)-4-hydroxyoxolan-2-yl]methoxy-hydroxyphosphoryl] phosphono hydrogen phosphate |
InChI |
InChI=1S/C20H28N10O20P4/c21-14-8-15(24-3-23-14)29(4-25-8)19-12(33)13(7(47-19)2-45-53(40,41)50-54(42,43)49-51(35,36)37)48-52(38,39)44-1-6-10(31)11(32)18(46-6)30-5-26-9-16(30)27-20(22)28-17(9)34/h3-7,10-13,18-19,31-33H,1-2H2,(H,38,39)(H,40,41)(H,42,43)(H2,21,23,24)(H2,35,36,37)(H3,22,27,28,34)/t6-,7-,10-,11-,12-,13-,18-,19-/m1/s1 |
InChI-Schlüssel |
DTYOICBSSHTIFB-INFSMZHSSA-N |
Isomerische SMILES |
C1=NC(=C2C(=N1)N(C=N2)[C@H]3[C@@H]([C@@H]([C@H](O3)COP(=O)(O)OP(=O)(O)OP(=O)(O)O)OP(=O)(O)OC[C@@H]4[C@H]([C@H]([C@@H](O4)N5C=NC6=C5N=C(NC6=O)N)O)O)O)N |
Kanonische SMILES |
C1=NC(=C2C(=N1)N(C=N2)C3C(C(C(O3)COP(=O)(O)OP(=O)(O)OP(=O)(O)O)OP(=O)(O)OCC4C(C(C(O4)N5C=NC6=C5N=C(NC6=O)N)O)O)O)N |
Herkunft des Produkts |
United States |
Haftungsausschluss und Informationen zu In-Vitro-Forschungsprodukten
Bitte beachten Sie, dass alle Artikel und Produktinformationen, die auf BenchChem präsentiert werden, ausschließlich zu Informationszwecken bestimmt sind. Die auf BenchChem zum Kauf angebotenen Produkte sind speziell für In-vitro-Studien konzipiert, die außerhalb lebender Organismen durchgeführt werden. In-vitro-Studien, abgeleitet von dem lateinischen Begriff "in Glas", beinhalten Experimente, die in kontrollierten Laborumgebungen unter Verwendung von Zellen oder Geweben durchgeführt werden. Es ist wichtig zu beachten, dass diese Produkte nicht als Arzneimittel oder Medikamente eingestuft sind und keine Zulassung der FDA für die Vorbeugung, Behandlung oder Heilung von medizinischen Zuständen, Beschwerden oder Krankheiten erhalten haben. Wir müssen betonen, dass jede Form der körperlichen Einführung dieser Produkte in Menschen oder Tiere gesetzlich strikt untersagt ist. Es ist unerlässlich, sich an diese Richtlinien zu halten, um die Einhaltung rechtlicher und ethischer Standards in Forschung und Experiment zu gewährleisten.