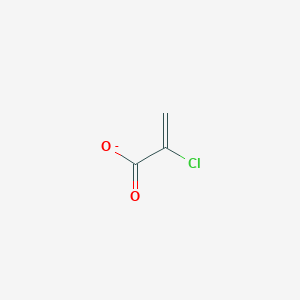
2-Chloroacrylate
- Klicken Sie auf QUICK INQUIRY, um ein Angebot von unserem Expertenteam zu erhalten.
- Mit qualitativ hochwertigen Produkten zu einem WETTBEWERBSFÄHIGEN Preis können Sie sich mehr auf Ihre Forschung konzentrieren.
Übersicht
Beschreibung
2-chloroacrylate is a monocarboxylic acid anion that is the conjugate base of 2-chloroacrylic acid obtained by deprotonation of the carboxy group. It has a role as a nitric oxide synthase activator. It is a conjugate base of a 2-chloroacrylic acid.
Wissenschaftliche Forschungsanwendungen
Biodegradation and Environmental Applications
A study by Kurata et al. (2005) discovered a novel enzyme, 2-haloacrylate reductase, in a soil bacterium Burkholderia sp. WS, which degrades 2-chloroacrylate. This enzyme catalyzes the reduction of the carbon-carbon double bond in this compound to produce (S)-2-chloropropionate, indicating a new pathway for the degradation of unsaturated organohalogen compounds (Kurata, Kurihara, Kamachi, & Esaki, 2005).
Industrial Production
Kurata et al. (2008) also developed a system for the asymmetric reduction of this compound to produce (S)-2-chloropropionate, a synthetic intermediate for phenoxypropionic acid herbicides. This system utilized recombinant Escherichia coli cells and achieved a high yield of (S)-2-chloropropionate in an enantioselective manner (Kurata, Fujita, Mowafy, Kamachi, Kurihara, & Esaki, 2008).
Polymer Science
Pathak et al. (1988) investigated the synthesis and copolymerization of 2-chloroethyl α-chloroacrylate. They studied its reactivity ratios and thermal properties, contributing to the understanding of copolymer behavior and properties (Pathak, Patni, & Babu, 1988).
Chemistry and Catalysis
Huang and Sun (2000) explored the chloropalladation carbonylation reaction of acetylenic selenides, leading to 2-seleno-3-chloroacrylates. Their work provided insights into regio- and stereoselectivity in organic synthesis, particularly for the production of chloroacrylates (Huang & Sun, 2000).
Eigenschaften
Molekularformel |
C3H2ClO2- |
---|---|
Molekulargewicht |
105.5 g/mol |
IUPAC-Name |
2-chloroprop-2-enoate |
InChI |
InChI=1S/C3H3ClO2/c1-2(4)3(5)6/h1H2,(H,5,6)/p-1 |
InChI-Schlüssel |
SZTBMYHIYNGYIA-UHFFFAOYSA-M |
SMILES |
C=C(C(=O)[O-])Cl |
Kanonische SMILES |
C=C(C(=O)[O-])Cl |
Synonyme |
2-chloroacrylate 2-chloroacrylic acid |
Herkunft des Produkts |
United States |
Retrosynthesis Analysis
AI-Powered Synthesis Planning: Our tool employs the Template_relevance Pistachio, Template_relevance Bkms_metabolic, Template_relevance Pistachio_ringbreaker, Template_relevance Reaxys, Template_relevance Reaxys_biocatalysis model, leveraging a vast database of chemical reactions to predict feasible synthetic routes.
One-Step Synthesis Focus: Specifically designed for one-step synthesis, it provides concise and direct routes for your target compounds, streamlining the synthesis process.
Accurate Predictions: Utilizing the extensive PISTACHIO, BKMS_METABOLIC, PISTACHIO_RINGBREAKER, REAXYS, REAXYS_BIOCATALYSIS database, our tool offers high-accuracy predictions, reflecting the latest in chemical research and data.
Strategy Settings
Precursor scoring | Relevance Heuristic |
---|---|
Min. plausibility | 0.01 |
Model | Template_relevance |
Template Set | Pistachio/Bkms_metabolic/Pistachio_ringbreaker/Reaxys/Reaxys_biocatalysis |
Top-N result to add to graph | 6 |
Feasible Synthetic Routes
Haftungsausschluss und Informationen zu In-Vitro-Forschungsprodukten
Bitte beachten Sie, dass alle Artikel und Produktinformationen, die auf BenchChem präsentiert werden, ausschließlich zu Informationszwecken bestimmt sind. Die auf BenchChem zum Kauf angebotenen Produkte sind speziell für In-vitro-Studien konzipiert, die außerhalb lebender Organismen durchgeführt werden. In-vitro-Studien, abgeleitet von dem lateinischen Begriff "in Glas", beinhalten Experimente, die in kontrollierten Laborumgebungen unter Verwendung von Zellen oder Geweben durchgeführt werden. Es ist wichtig zu beachten, dass diese Produkte nicht als Arzneimittel oder Medikamente eingestuft sind und keine Zulassung der FDA für die Vorbeugung, Behandlung oder Heilung von medizinischen Zuständen, Beschwerden oder Krankheiten erhalten haben. Wir müssen betonen, dass jede Form der körperlichen Einführung dieser Produkte in Menschen oder Tiere gesetzlich strikt untersagt ist. Es ist unerlässlich, sich an diese Richtlinien zu halten, um die Einhaltung rechtlicher und ethischer Standards in Forschung und Experiment zu gewährleisten.