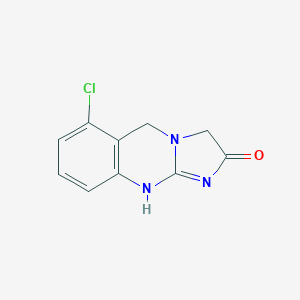
Dechloro Anagrelid
Übersicht
Beschreibung
Dechloro Anagrelide is an analogue of Anagrelide, A phosphodiesterase inhibitor with antiplatelet activity. Used as an antithrombocythemic.
Wissenschaftliche Forschungsanwendungen
Krebstherapie
Anagrelid, ein etabliertes Medikament zur Senkung der Thrombozytenzahl, hat eine neue Verwendung als unterstützende Therapie in der Krebstherapie gefunden . Es wurde festgestellt, dass Anagrelid die von Krebszellen stimulierte Thrombozytenbildung blockieren und die Migration von Krebszellen zu Thrombozytenvorläufern hemmen kann . Dies hat das Potenzial, die Überlebensraten bei Krebs aus einer Reihe von soliden Tumoren zu verbessern .
Thrombozytose-Behandlung
Anagrelid wird seit über zwanzig Jahren in den USA und Europa als Behandlung für Thrombozytose vermarktet . Thrombozytose ist ein Zustand, der durch extrem hohe Thrombozytenzahlen gekennzeichnet ist, die zu thrombotischen (gerinnungsbedingten) Episoden führen können . Anagrelid senkt das Risiko dieser Episoden .
Rolle bei der Krebsentwicklung
Die Forschung hat gezeigt, dass Thrombozyten, die normalerweise mit der Blutgerinnung in Verbindung gebracht werden, auch an der Krebsentwicklung beteiligt sind . Anagrelid kann an mehreren Stellen in diesem Prozess wirken .
Paraneoplastische Thrombozytose
Bei Krebspatienten mit Thrombozytose wird die erhöhte Thrombozytenzahl oft direkt durch den Krebs verursacht . Dieser Zustand wird als "paraneoplastische Thrombozytose" bezeichnet
Wirkmechanismus
Target of Action
Dechloro Anagrelide primarily targets platelets . It is used as a platelet-reducing agent to lower dangerously elevated platelet levels, particularly in patients with myeloproliferative neoplasms .
Mode of Action
Dechloro Anagrelide works by inhibiting the maturation of platelets from megakaryocytes . . It is a potent inhibitor of phosphodiesterase-II . It also inhibits PDE-3 and phospholipase A2 .
Biochemical Pathways
Dechloro Anagrelide affects the biochemical pathways related to platelet production. It suppresses transcription factors necessary for the synthesis and maturation of platelet-producing cells . This leads to a decrease in platelet counts . It also interferes with platelet-activating signal pathways and blocks intracellular signaling networks, rearrangements, and fibrinogen receptor activation .
Pharmacokinetics
Dechloro Anagrelide is extensively metabolized, primarily in the liver by cytochrome P450 1A2 (CYP1A2), into two major metabolites: 6,7-dichloro-3-hydroxy-1,5 dihydro-imidazo[2,1-b]quinazolin-2-one (3-hydroxy anagrelide) and 2-amino-5,6-dichloro-3,4,-dihydroquinazoline (RL603) . The pharmacokinetics of Dechloro Anagrelide can be influenced by factors such as food intake . For instance, food has been shown to increase the bioavailability of Anagrelide .
Result of Action
The molecular and cellular effects of Dechloro Anagrelide’s action primarily involve a reduction in platelet counts. This is achieved through a gradual suppression of platelet-producing cells . It may take 7 to 14 days for its administration to be reflected in reduced platelet counts .
Action Environment
The action, efficacy, and stability of Dechloro Anagrelide can be influenced by various environmental factors. For instance, the drug’s pharmacokinetics and thus its action can be affected by factors such as food intake . Furthermore, the drug’s action can also be influenced by the patient’s specific physiological environment, including liver function due to the role of liver enzymes in the drug’s metabolism .
Biochemische Analyse
Biochemical Properties
Dechloro Anagrelide interacts with various biomolecules in the body. It inhibits platelet function by inhibiting a type 3 phosphodiesterase . This inhibition leads to an increase in cyclic adenosine monophosphate (cAMP), which interferes with platelet-activating signal pathways and blocks signaling networks .
Cellular Effects
Dechloro Anagrelide has significant effects on various types of cells and cellular processes. It rapidly lowers platelet counts . In cell culture, it inhibits the maturation of megakaryocytes, thereby reducing their size and ploidy .
Molecular Mechanism
The mechanism of action of Dechloro Anagrelide involves its interaction with biomolecules at the molecular level. It inhibits platelet function by inhibiting a type 3 phosphodiesterase . This leads to a subsequent increase in cAMP . Dechloro Anagrelide also inhibits the release of arachidonic acid metabolites from human platelets after stimulation with thrombin .
Temporal Effects in Laboratory Settings
The effects of Dechloro Anagrelide change over time in laboratory settings. Due to its short half-life, an increase overshoot is observed about 4 to 8 days after discontinuation of the drug .
Dosage Effects in Animal Models
The effects of Dechloro Anagrelide vary with different dosages in animal models. For instance, oral doses of 1, 2.5, and 10 mg/kg reduced thrombus mass in a biolaser-induced thrombus formation model in the capillary vessels of the ear of a lop-eared rabbit .
Metabolic Pathways
Dechloro Anagrelide is extensively metabolized by the liver into two major metabolites
Eigenschaften
IUPAC Name |
6-chloro-3,5-dihydro-1H-imidazo[2,1-b]quinazolin-2-one | |
---|---|---|
Source | PubChem | |
URL | https://pubchem.ncbi.nlm.nih.gov | |
Description | Data deposited in or computed by PubChem | |
InChI |
InChI=1S/C10H8ClN3O/c11-7-2-1-3-8-6(7)4-14-5-9(15)13-10(14)12-8/h1-3H,4-5H2,(H,12,13,15) | |
Source | PubChem | |
URL | https://pubchem.ncbi.nlm.nih.gov | |
Description | Data deposited in or computed by PubChem | |
InChI Key |
RFUZAPDLTCFPQE-UHFFFAOYSA-N | |
Source | PubChem | |
URL | https://pubchem.ncbi.nlm.nih.gov | |
Description | Data deposited in or computed by PubChem | |
Canonical SMILES |
C1C2=C(C=CC=C2Cl)N=C3N1CC(=O)N3 | |
Source | PubChem | |
URL | https://pubchem.ncbi.nlm.nih.gov | |
Description | Data deposited in or computed by PubChem | |
Molecular Formula |
C10H8ClN3O | |
Source | PubChem | |
URL | https://pubchem.ncbi.nlm.nih.gov | |
Description | Data deposited in or computed by PubChem | |
Molecular Weight |
221.64 g/mol | |
Source | PubChem | |
URL | https://pubchem.ncbi.nlm.nih.gov | |
Description | Data deposited in or computed by PubChem | |
Retrosynthesis Analysis
AI-Powered Synthesis Planning: Our tool employs the Template_relevance Pistachio, Template_relevance Bkms_metabolic, Template_relevance Pistachio_ringbreaker, Template_relevance Reaxys, Template_relevance Reaxys_biocatalysis model, leveraging a vast database of chemical reactions to predict feasible synthetic routes.
One-Step Synthesis Focus: Specifically designed for one-step synthesis, it provides concise and direct routes for your target compounds, streamlining the synthesis process.
Accurate Predictions: Utilizing the extensive PISTACHIO, BKMS_METABOLIC, PISTACHIO_RINGBREAKER, REAXYS, REAXYS_BIOCATALYSIS database, our tool offers high-accuracy predictions, reflecting the latest in chemical research and data.
Strategy Settings
Precursor scoring | Relevance Heuristic |
---|---|
Min. plausibility | 0.01 |
Model | Template_relevance |
Template Set | Pistachio/Bkms_metabolic/Pistachio_ringbreaker/Reaxys/Reaxys_biocatalysis |
Top-N result to add to graph | 6 |
Feasible Synthetic Routes
Haftungsausschluss und Informationen zu In-Vitro-Forschungsprodukten
Bitte beachten Sie, dass alle Artikel und Produktinformationen, die auf BenchChem präsentiert werden, ausschließlich zu Informationszwecken bestimmt sind. Die auf BenchChem zum Kauf angebotenen Produkte sind speziell für In-vitro-Studien konzipiert, die außerhalb lebender Organismen durchgeführt werden. In-vitro-Studien, abgeleitet von dem lateinischen Begriff "in Glas", beinhalten Experimente, die in kontrollierten Laborumgebungen unter Verwendung von Zellen oder Geweben durchgeführt werden. Es ist wichtig zu beachten, dass diese Produkte nicht als Arzneimittel oder Medikamente eingestuft sind und keine Zulassung der FDA für die Vorbeugung, Behandlung oder Heilung von medizinischen Zuständen, Beschwerden oder Krankheiten erhalten haben. Wir müssen betonen, dass jede Form der körperlichen Einführung dieser Produkte in Menschen oder Tiere gesetzlich strikt untersagt ist. Es ist unerlässlich, sich an diese Richtlinien zu halten, um die Einhaltung rechtlicher und ethischer Standards in Forschung und Experiment zu gewährleisten.