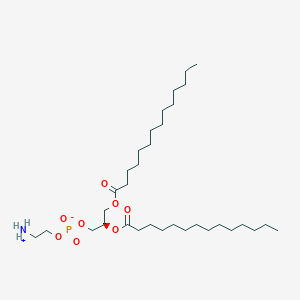
1,2-Dimyristoyl-sn-glycero-3-phosphoethanolamine
Übersicht
Beschreibung
DMPE is a water-soluble derivative of phosphatidylethanolamine with (14:0) myristic acid acyl chains . It is also used in proteoliposomes for planar supported lipid bilayer formation .
Synthesis Analysis
DMPE is a synthetic phospholipid . It is formed primarily in the reaction of CDP-ethanolamine and diacylglycerol .Molecular Structure Analysis
The molecular formula of DMPE is C33H66NO8P . The molecular weight and exact mass are averages based on the polydispersity of PEG .Chemical Reactions Analysis
DMPE is commonly used in the generation of micelles, liposomes, and other types of artificial membranes . It has been used as a standard for phospholipid analysis by LC-ESI-MS .Physical And Chemical Properties Analysis
DMPE is a solid at room temperature . It is soluble in water (partly), and CHCl3:MeOH (4:1) (50 mg/ml) .Wissenschaftliche Forschungsanwendungen
- Application : Researchers utilize DMPE to create liposomes that encapsulate therapeutic agents, allowing targeted drug delivery to specific tissues or cells .
- Application : DMPE contributes to the formation of SLBs, providing a platform for investigating membrane-associated processes, such as protein-lipid interactions and membrane dynamics .
- Application : DMPE is a key component in bicelles, enabling the study of membrane proteins and their interactions in a more native-like environment .
- Application : Researchers use DMPE as a reference compound to quantify other lipids or analytes in complex mixtures .
- Application : DMPE is incorporated into FFs, allowing researchers to investigate diffusion behavior and interactions at lipid interfaces .
Liposome Formation and Drug Delivery
Planar Supported Lipid Bilayers (SLBs)
Bicelles for Structural Studies
Internal Standard in Chromatography
Foam Films (FFs) for Diffusion Studies
Studying Phospholipid Signaling Pathways
Wirkmechanismus
Target of Action
1,2-Dimyristoyl-sn-glycero-3-phosphoethanolamine (DMPE) is a lipid found in the cell membranes of bacteria and human nervous tissues . It primarily targets cell membranes due to its similar phospholipid structures .
Mode of Action
DMPE interacts with its targets, the cell membranes, by integrating into the lipid bilayer. This interaction can alter the physical properties of the membrane, such as its fluidity and permeability . DMPE has been shown to enhance the constancy and in vitro antiproliferative effect of certain drugs .
Biochemical Pathways
DMPE affects the biochemical pathways related to membrane function and integrity. By integrating into the lipid bilayer, it can influence membrane-associated processes, such as signal transduction and substance transport .
Result of Action
The integration of DMPE into cell membranes can result in changes to the membrane’s physical properties, potentially affecting the function of membrane-bound proteins and the cell’s overall behavior . In some cases, DMPE has been shown to enhance the constancy and in vitro antiproliferative effect of certain drugs .
Action Environment
The action of DMPE can be influenced by various environmental factors. For instance, the presence of other lipids can affect its integration into cell membranes . Additionally, factors such as pH and temperature can influence the stability and efficacy of DMPE .
Zukünftige Richtungen
Eigenschaften
IUPAC Name |
[(2R)-3-[2-aminoethoxy(hydroxy)phosphoryl]oxy-2-tetradecanoyloxypropyl] tetradecanoate | |
---|---|---|
Source | PubChem | |
URL | https://pubchem.ncbi.nlm.nih.gov | |
Description | Data deposited in or computed by PubChem | |
InChI |
InChI=1S/C33H66NO8P/c1-3-5-7-9-11-13-15-17-19-21-23-25-32(35)39-29-31(30-41-43(37,38)40-28-27-34)42-33(36)26-24-22-20-18-16-14-12-10-8-6-4-2/h31H,3-30,34H2,1-2H3,(H,37,38)/t31-/m1/s1 | |
Source | PubChem | |
URL | https://pubchem.ncbi.nlm.nih.gov | |
Description | Data deposited in or computed by PubChem | |
InChI Key |
NEZDNQCXEZDCBI-WJOKGBTCSA-N | |
Source | PubChem | |
URL | https://pubchem.ncbi.nlm.nih.gov | |
Description | Data deposited in or computed by PubChem | |
Canonical SMILES |
CCCCCCCCCCCCCC(=O)OCC(COP(=O)(O)OCCN)OC(=O)CCCCCCCCCCCCC | |
Source | PubChem | |
URL | https://pubchem.ncbi.nlm.nih.gov | |
Description | Data deposited in or computed by PubChem | |
Isomeric SMILES |
CCCCCCCCCCCCCC(=O)OC[C@H](COP(=O)(O)OCCN)OC(=O)CCCCCCCCCCCCC | |
Source | PubChem | |
URL | https://pubchem.ncbi.nlm.nih.gov | |
Description | Data deposited in or computed by PubChem | |
Molecular Formula |
C33H66NO8P | |
Source | PubChem | |
URL | https://pubchem.ncbi.nlm.nih.gov | |
Description | Data deposited in or computed by PubChem | |
DSSTOX Substance ID |
DTXSID601281409 | |
Record name | Dimyristoylphosphatidylethanolamine | |
Source | EPA DSSTox | |
URL | https://comptox.epa.gov/dashboard/DTXSID601281409 | |
Description | DSSTox provides a high quality public chemistry resource for supporting improved predictive toxicology. | |
Molecular Weight |
635.9 g/mol | |
Source | PubChem | |
URL | https://pubchem.ncbi.nlm.nih.gov | |
Description | Data deposited in or computed by PubChem | |
Physical Description |
Solid | |
Record name | PE(14:0/14:0) | |
Source | Human Metabolome Database (HMDB) | |
URL | http://www.hmdb.ca/metabolites/HMDB0008821 | |
Description | The Human Metabolome Database (HMDB) is a freely available electronic database containing detailed information about small molecule metabolites found in the human body. | |
Explanation | HMDB is offered to the public as a freely available resource. Use and re-distribution of the data, in whole or in part, for commercial purposes requires explicit permission of the authors and explicit acknowledgment of the source material (HMDB) and the original publication (see the HMDB citing page). We ask that users who download significant portions of the database cite the HMDB paper in any resulting publications. | |
CAS RN |
998-07-2 | |
Record name | (R)-Dimyristoylphosphatidylethanolamine | |
Source | CAS Common Chemistry | |
URL | https://commonchemistry.cas.org/detail?cas_rn=998-07-2 | |
Description | CAS Common Chemistry is an open community resource for accessing chemical information. Nearly 500,000 chemical substances from CAS REGISTRY cover areas of community interest, including common and frequently regulated chemicals, and those relevant to high school and undergraduate chemistry classes. This chemical information, curated by our expert scientists, is provided in alignment with our mission as a division of the American Chemical Society. | |
Explanation | The data from CAS Common Chemistry is provided under a CC-BY-NC 4.0 license, unless otherwise stated. | |
Record name | 1,2-Dimyristoyl-sn-glycero-3-phosphoethanolamine | |
Source | ChemIDplus | |
URL | https://pubchem.ncbi.nlm.nih.gov/substance/?source=chemidplus&sourceid=0000998072 | |
Description | ChemIDplus is a free, web search system that provides access to the structure and nomenclature authority files used for the identification of chemical substances cited in National Library of Medicine (NLM) databases, including the TOXNET system. | |
Record name | Dimyristoylphosphatidylethanolamine | |
Source | EPA DSSTox | |
URL | https://comptox.epa.gov/dashboard/DTXSID601281409 | |
Description | DSSTox provides a high quality public chemistry resource for supporting improved predictive toxicology. | |
Record name | 1,2-DIMYRISTOYL-SN-GLYCERO-3-PHOSPHOETHANOLAMINE | |
Source | FDA Global Substance Registration System (GSRS) | |
URL | https://gsrs.ncats.nih.gov/ginas/app/beta/substances/Z37OX1ASNK | |
Description | The FDA Global Substance Registration System (GSRS) enables the efficient and accurate exchange of information on what substances are in regulated products. Instead of relying on names, which vary across regulatory domains, countries, and regions, the GSRS knowledge base makes it possible for substances to be defined by standardized, scientific descriptions. | |
Explanation | Unless otherwise noted, the contents of the FDA website (www.fda.gov), both text and graphics, are not copyrighted. They are in the public domain and may be republished, reprinted and otherwise used freely by anyone without the need to obtain permission from FDA. Credit to the U.S. Food and Drug Administration as the source is appreciated but not required. | |
Record name | PE(14:0/14:0) | |
Source | Human Metabolome Database (HMDB) | |
URL | http://www.hmdb.ca/metabolites/HMDB0008821 | |
Description | The Human Metabolome Database (HMDB) is a freely available electronic database containing detailed information about small molecule metabolites found in the human body. | |
Explanation | HMDB is offered to the public as a freely available resource. Use and re-distribution of the data, in whole or in part, for commercial purposes requires explicit permission of the authors and explicit acknowledgment of the source material (HMDB) and the original publication (see the HMDB citing page). We ask that users who download significant portions of the database cite the HMDB paper in any resulting publications. | |
Retrosynthesis Analysis
AI-Powered Synthesis Planning: Our tool employs the Template_relevance Pistachio, Template_relevance Bkms_metabolic, Template_relevance Pistachio_ringbreaker, Template_relevance Reaxys, Template_relevance Reaxys_biocatalysis model, leveraging a vast database of chemical reactions to predict feasible synthetic routes.
One-Step Synthesis Focus: Specifically designed for one-step synthesis, it provides concise and direct routes for your target compounds, streamlining the synthesis process.
Accurate Predictions: Utilizing the extensive PISTACHIO, BKMS_METABOLIC, PISTACHIO_RINGBREAKER, REAXYS, REAXYS_BIOCATALYSIS database, our tool offers high-accuracy predictions, reflecting the latest in chemical research and data.
Strategy Settings
Precursor scoring | Relevance Heuristic |
---|---|
Min. plausibility | 0.01 |
Model | Template_relevance |
Template Set | Pistachio/Bkms_metabolic/Pistachio_ringbreaker/Reaxys/Reaxys_biocatalysis |
Top-N result to add to graph | 6 |
Feasible Synthetic Routes
Q & A
Q1: How does 1,2-Dimyristoyl-sn-glycero-3-phosphoethanolamine (DMPE) interact with antimicrobial peptides (AMPs)?
A: AMPs can insert themselves into DMPE bilayers to varying degrees, influencing the dynamics of the phospholipids. [] The interaction appears to be stronger with DMPE than with phosphatidylcholine (PC) lipids, leading to increased destabilization and morphological changes in DMPE vesicles upon AMP addition. []
Q2: What is the role of DMPE in bacterial membranes and its interaction with host defense peptides?
A: DMPE is a significant component of bacterial membranes. Research indicates that some host defense peptides, like Maximin H5, exhibit a higher binding affinity for DMPE-rich membranes. [] This interaction can limit the peptide's ability to lyse the bacterial membrane, potentially contributing to bacterial resistance against host defense mechanisms. []
Q3: Can the interaction between DMPE and cholesterol impact membrane properties?
A: Yes, nearest-neighbor recognition experiments suggest that cholesterol's condensing effect on phospholipid bilayers is primarily observed in the liquid-ordered phase. [] This implies that the presence of cholesterol can significantly influence the packing and organization of DMPE molecules within the membrane. []
Q4: What is the molecular formula and weight of DMPE?
A4: The molecular formula of DMPE is C36H72NO8P, and its molecular weight is 637.9 g/mol.
Q5: How does the chain length of phospholipids in a mixture affect the permeability of the resulting bilayers?
A: Studies using liposomes made from mixtures of DMPE and 1,2-distearoyl-sn-glycero-3-phosphocholine (DSPC) show that the permeability of the bilayer is affected by the composition of the lipid phases at the boundaries between liquid crystal and gel states. [] This suggests that DMPE may segregate at these boundaries, influencing the overall permeability of the membrane. []
Q6: How does DMPE behave in mixed monolayers with other phospholipids?
A: DMPE exhibits different miscibility behaviors with various phospholipids. While it shows limited miscibility with phosphoglycerol (PG)-like lipids in the fluid bilayer state, [] it displays non-ideal mixing behavior with 1,2-distearoyl-sn-glycero-3-phosphoethanolamine (DSPE) in the presence of certain diluents like 1,2-dilauroyl-sn-glycero-3-phosphocholine (DLPC). []
Q7: What is the impact of temperature on the organization of water molecules around DMPE bilayers?
A: Research using Small Angle X-ray Scattering (SAXS) and densitometry revealed that the water layer surrounding DMPE bilayers, particularly the "perturbed water" layer, expands with increasing temperature. [] This indicates a temperature-dependent interaction between DMPE and water molecules at the membrane interface. []
Q8: Does the presence of cholesterol affect the interaction between DMPE and water?
A: Studies utilizing nearest-neighbor recognition experiments with exchangeable DMPE dimers suggest that replacing water (H2O) with deuterium oxide (D2O) impacts DMPE mixing only in saturated bilayers lacking cholesterol. [] This indicates that cholesterol may influence the hydration properties of DMPE-containing membranes. []
A8: This section is not applicable as DMPE is primarily a structural lipid and not known to possess inherent catalytic properties.
Q9: How can molecular dynamics simulations be employed to understand DMPE interactions?
A: Molecular dynamics simulations can offer valuable insights into the behavior of DMPE within biological systems. For instance, simulations have been used to examine the stability of myelin basic protein (MBP) on mixed DMPC/DMPE bilayers. [] These simulations revealed that the composition of the lipid bilayer influences MBP's interaction with the membrane, providing insights into the structural dynamics of myelin. []
Q10: Can molecular modeling help understand the interaction of drug conjugates with DMPE membranes?
A: Yes, molecular modeling techniques, in conjunction with experimental methods like Langmuir monolayer studies, can provide insights into how drug conjugates interact with DMPE-based model bacterial membranes. [] This approach can reveal details about the binding modes, conformational changes, and potential impact on membrane hydration. []
Q11: How does the linkage region of sphingolipids influence their mixing with DMPE in cholesterol-rich environments?
A: Nearest-neighbor recognition studies suggest that the linkage region of sphingolipids plays a crucial role in their interaction with phospholipids like DMPE, especially in cholesterol-rich bilayers. [] The presence of cholesterol enhances the preference for homolipid association, indicating that the sphingolipid's linkage region contributes to its segregation from DMPE within the membrane. []
Q12: How does the bridging group in exchangeable DMPE dimers influence nearest-neighbor recognition (NNR) within fluid bilayers?
A: Research indicates that the length and chemical nature of the bridging group in DMPE dimers can significantly impact NNR within fluid bilayers. [] Longer bridging groups tend to enhance NNR, suggesting that they influence the packing and organization of DMPE molecules. [] Cholesterol's effect on NNR was also found to be dependent on the bridging group length. []
Q13: How stable are liposomes composed of DMPE, dihexadecyl phosphate (DCP), and cholesterol?
A: Liposomes with varying ratios of DMPE, DCP, and cholesterol have been studied for their physicochemical properties and biological activity. [] Results suggest that these liposomes are stable at physiological temperatures, making them suitable for in vivo applications. [] The addition of DCP appears to improve colloidal stability. []
A13: Specific SHE regulations are not explicitly discussed in the provided research papers.
A13: The provided research focuses mainly on the biophysical properties and interactions of DMPE rather than its pharmacokinetic and pharmacodynamic aspects.
Q14: How effective are liposomes containing DMPE in delivering therapeutic agents?
A: Studies have investigated the efficacy of DMPE-containing liposomes as delivery systems for therapeutic agents. Liposomes prepared with DMPE and DCP showed the best efficiency in an in vitro model mimicking in vivo release of bioactive substances. []
Q15: How does the presence of DMPE in bacterial membranes contribute to bacterial resistance?
A: The presence of DMPE in bacterial membranes can contribute to resistance against certain antimicrobial agents, such as host defense peptides. [] The specific interaction between DMPE and these peptides can hinder their membrane-disrupting activity, allowing bacteria to evade the immune response. []
A15: Toxicity and safety data specific to DMPE are not explicitly detailed in the provided research papers.
Q16: Can hyaluronic acid-decorated liposomes be used for targeted drug delivery to the skin?
A: Research suggests that hyaluronic acid-decorated liposomes, particularly those incorporating DMPE as a lipid anchor, hold promise for targeted drug delivery to the skin. [] The deformability of these liposomes and the extent of hyaluronic acid conjugation appear to be crucial factors influencing skin penetration. []
Q17: What is the potential of lipid nanoparticles containing DMPE for siRNA delivery to immune cells?
A: Lipid nanoparticles composed of a novel cationic lipid, DOPE, and PEG2000-DMPE have shown potential for siRNA delivery to immune cells like macrophages and dendritic cells. [] These nanoparticles demonstrated efficient siRNA encapsulation and successful gene knockdown in both in vitro and in vivo models. []
A17: Specific biomarkers and diagnostics related to DMPE are not directly addressed in the provided research papers.
Q18: What are some analytical techniques used to study DMPE-containing systems?
A18: Various techniques have been employed to characterize and study DMPE-containing systems. Some examples include:
- Langmuir monolayer studies: These studies provide insights into the interactions of DMPE with other molecules at the air-water interface. [, , ]
- Atomic force microscopy (AFM): AFM can be used to visualize the morphology and organization of DMPE molecules within model membranes. []
- Neutron reflectometry: This technique helps determine the structure and thickness of DMPE-containing layers within multilayered films. []
- Quasi-elastic neutron scattering (QENS): QENS provides information about the dynamics of DMPE molecules and associated water molecules within bilayers. []
- Polarization modulation infrared reflection-absorption spectroscopy (PM-IRRAS): PM-IRRAS offers insights into the orientation and conformation of DMPE molecules within monolayers. []
- Brewster angle microscopy (BAM): BAM visualizes the morphology and phase behavior of DMPE monolayers at the air-water interface. [, ]
- Differential scanning calorimetry (DSC): DSC measures the thermal transitions of DMPE-containing systems, providing insights into their phase behavior. [, , ]
- Dynamic light scattering (DLS): DLS measures the size and diffusion properties of DMPE-containing liposomes and vesicles. [, , , ]
- Small angle X-ray/neutron scattering (SAXS/SANS): SAXS and SANS provide structural information about DMPE-containing bilayers and vesicles. [, , ]
- Time-resolved small-angle neutron scattering (TR-SANS): TR-SANS investigates the dynamics of lipid flip-flop and exchange within DMPE-containing vesicles. [, ]
Haftungsausschluss und Informationen zu In-Vitro-Forschungsprodukten
Bitte beachten Sie, dass alle Artikel und Produktinformationen, die auf BenchChem präsentiert werden, ausschließlich zu Informationszwecken bestimmt sind. Die auf BenchChem zum Kauf angebotenen Produkte sind speziell für In-vitro-Studien konzipiert, die außerhalb lebender Organismen durchgeführt werden. In-vitro-Studien, abgeleitet von dem lateinischen Begriff "in Glas", beinhalten Experimente, die in kontrollierten Laborumgebungen unter Verwendung von Zellen oder Geweben durchgeführt werden. Es ist wichtig zu beachten, dass diese Produkte nicht als Arzneimittel oder Medikamente eingestuft sind und keine Zulassung der FDA für die Vorbeugung, Behandlung oder Heilung von medizinischen Zuständen, Beschwerden oder Krankheiten erhalten haben. Wir müssen betonen, dass jede Form der körperlichen Einführung dieser Produkte in Menschen oder Tiere gesetzlich strikt untersagt ist. Es ist unerlässlich, sich an diese Richtlinien zu halten, um die Einhaltung rechtlicher und ethischer Standards in Forschung und Experiment zu gewährleisten.