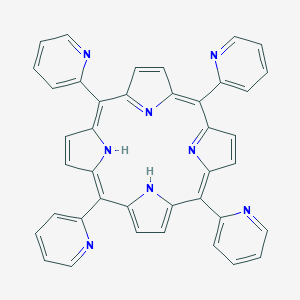
5,10,15,20-Tetrakis(2-pyridyl)porphyrin
Übersicht
Beschreibung
5,10,15,20-Tetrakis(2-pyridyl)porphyrin, also known as this compound, is a useful research compound. Its molecular formula is C40H26N8 and its molecular weight is 618.7 g/mol. The purity is usually 95%.
BenchChem offers high-quality this compound suitable for many research applications. Different packaging options are available to accommodate customers' requirements. Please inquire for more information about this compound including the price, delivery time, and more detailed information at info@benchchem.com.
Wissenschaftliche Forschungsanwendungen
Photokatalyse
5,10,15,20-Tetrakis(2-pyridyl)porphyrin: wurde in der Photokatalyse eingesetzt, da es Lichtenergie nutzen kann, um chemische Reaktionen anzutreiben. Der Porphyrinkomplex mit Sn(IV) und trans-Dihydroxo-Axialliganden zeigt interessante Funktionen, wenn er in photokatalytischen Prozessen eingesetzt wird . Dazu gehören der Abbau von Schadstoffen und die Wasserspaltung zur Wasserstoffproduktion.
Biomedizin
Das Potenzial der Verbindung in der Biomedizin ist bedeutend. Es wurde für die Verwendung in der Photodynamischen Therapie untersucht, bei der es durch Licht aktiviert werden kann, um reaktive Sauerstoffspezies zu erzeugen, die Krebszellen oder Krankheitserreger abtöten können . Diese Anwendung nutzt die starke Absorption der Verbindung im sichtbaren Bereich und ihre Fähigkeit, Singulett-Sauerstoff zu erzeugen.
Arzneimittelentwicklung
Kationische Derivate von This compound wurden synthetisiert, wobei ihre Verwendung als Medikamente zur Behandlung von Erkrankungen wie ischämischer Herzkrankheit und Zerebralparese vorhergesagt wurde . Die Porphyrinstruktur ermöglicht die Interaktion mit biologischen Molekülen, die im Arzneimittel-Design genutzt werden können.
Materialwissenschaft
In der Materialwissenschaft wurde dieses Porphyrin verwendet, um Nanostrukturen und Multiporphyrin-Arrays aufgrund seiner zusätzlichen Bindungsstellen zu erzeugen . Diese Strukturen haben Anwendungen bei der Herstellung anspruchsvoller Materialien mit einzigartigen elektronischen, optischen und katalytischen Eigenschaften.
Umweltanwendungen
Die Verbindung wurde verwendet, um Montmorillonit, ein Tonmineral, zu modifizieren, um Verbundwerkstoffe mit verbesserten Adsorptionskapazitäten zu erzeugen . Dies ist besonders nützlich für die Adsorption von giftigen Substanzen, wodurch es zu einem wertvollen Material für Umweltbereinigungsmaßnahmen wird.
Supramolekulare Chemie
Das This compound spielt eine Rolle bei der Entwicklung der supramolekularen Chemie. Seine Fähigkeit, Wasserstoffbrückenbindungen zu bilden und sich durch diese Wechselwirkungen zu zweidimensionalen Netzwerken zusammenzulagern, ist entscheidend für die Herstellung größerer, funktionaler Strukturen .
Wirkmechanismus
Target of Action
5,10,15,20-Tetrakis(2-pyridyl)porphyrin, also known as 5,10,15,20-tetra(pyridin-2-yl)porphyrin, is a type of porphyrin that can coordinate transition metal ions such as iron, cobalt, and magnesium . These molecules are associated with proteins involved in various biological systems, including photosynthesis, cell respiration, cell death, and antioxidant defense .
Mode of Action
The mode of action of this compound is primarily through its interaction with its targets. For instance, in synthetic porphyrins such as this, the catalytic and photochemical properties can be achieved by the coordination with a diversity of central metal ions . The coordination of nitrogen to the central zinc (II) ion of another molecule is crucial for the formation of the fibers .
Biochemical Pathways
The biochemical pathways affected by this compound are diverse. In photodynamic therapy (PDT), this compound and other porphyrins act as photosensitizers . The photochemical properties of this compound are also useful for the fabrication of solar cells . The catalytic properties require the presence of a central metal .
Pharmacokinetics
It’s known that the compound is a biochemical reagent that can be used as a biological material or organic compound for life science-related research .
Result of Action
The molecular and cellular effects of this compound’s action are diverse. For instance, it has been shown to enhance the visible photocatalytic activity of certain nanoparticles in the degradation of methylene blue . It also exhibits an effective adsorption capacity for the removal of heavy metals such as Pb (II) and Cu (II) in an aqueous medium .
Action Environment
The action of this compound can be influenced by environmental factors. For instance, the surfactant-assisted synthetic route appears to provide a promising method for the construction of highly organized three-dimensional organic micro-structures of this compound . The presence of the surfactant was essential to make and to maintain the fibers .
Biochemische Analyse
Biochemical Properties
The electronic and spectroscopic properties of 5,10,15,20-Tetrakis(2-pyridyl)porphyrin were investigated in the framework of the density functional theory (DFT). Modeling of implicit solvent, charge effects, and medium acidity were performed and compared with experimental results . Porphyrins have some unique characteristics such as an intense adsorption band in the visible region, generally related to a π-π* transition, and redox properties which can be tuned by substituents exchange .
Cellular Effects
Systemic effects of this compound were investigated using Drosophila melanogaster adult males. It was found that this compound was absorbed and stored higher in neuronal than in non-neuronal extracts . This indicates that the compound can influence cell function by interacting with different cellular components.
Temporal Effects in Laboratory Settings
Twenty-four hours after oral administration of this compound, the quantity of hydrogen peroxide (H2O2) increased, but exclusively in the head extracts. Regardless of photoactivation, this compound resulted in a reduced concentration of H2O2 after 7 days .
Dosage Effects in Animal Models
The dosage effects of this compound in animal models have not been extensively studied. It has been shown that the compound can disrupt redox regulation, impairing cellular signaling and behavioral outcomes in the process .
Eigenschaften
IUPAC Name |
5,10,15,20-tetrapyridin-2-yl-21,22-dihydroporphyrin | |
---|---|---|
Details | Computed by Lexichem TK 2.7.0 (PubChem release 2021.05.07) | |
Source | PubChem | |
URL | https://pubchem.ncbi.nlm.nih.gov | |
Description | Data deposited in or computed by PubChem | |
InChI |
InChI=1S/C40H26N8/c1-5-21-41-25(9-1)37-29-13-15-31(45-29)38(26-10-2-6-22-42-26)33-17-19-35(47-33)40(28-12-4-8-24-44-28)36-20-18-34(48-36)39(27-11-3-7-23-43-27)32-16-14-30(37)46-32/h1-24,45-46H | |
Details | Computed by InChI 1.0.6 (PubChem release 2021.05.07) | |
Source | PubChem | |
URL | https://pubchem.ncbi.nlm.nih.gov | |
Description | Data deposited in or computed by PubChem | |
InChI Key |
MWOBSIASKLRDBM-UHFFFAOYSA-N | |
Details | Computed by InChI 1.0.6 (PubChem release 2021.05.07) | |
Source | PubChem | |
URL | https://pubchem.ncbi.nlm.nih.gov | |
Description | Data deposited in or computed by PubChem | |
Canonical SMILES |
C1=CC=NC(=C1)C2=C3C=CC(=C(C4=CC=C(N4)C(=C5C=CC(=N5)C(=C6C=CC2=N6)C7=CC=CC=N7)C8=CC=CC=N8)C9=CC=CC=N9)N3 | |
Details | Computed by OEChem 2.3.0 (PubChem release 2021.05.07) | |
Source | PubChem | |
URL | https://pubchem.ncbi.nlm.nih.gov | |
Description | Data deposited in or computed by PubChem | |
Molecular Formula |
C40H26N8 | |
Details | Computed by PubChem 2.1 (PubChem release 2021.05.07) | |
Source | PubChem | |
URL | https://pubchem.ncbi.nlm.nih.gov | |
Description | Data deposited in or computed by PubChem | |
DSSTOX Substance ID |
DTXSID10408775 | |
Record name | 5,10,15,20-tetrapyridin-2-yl-21,22-dihydroporphyrin | |
Source | EPA DSSTox | |
URL | https://comptox.epa.gov/dashboard/DTXSID10408775 | |
Description | DSSTox provides a high quality public chemistry resource for supporting improved predictive toxicology. | |
Molecular Weight |
618.7 g/mol | |
Details | Computed by PubChem 2.1 (PubChem release 2021.05.07) | |
Source | PubChem | |
URL | https://pubchem.ncbi.nlm.nih.gov | |
Description | Data deposited in or computed by PubChem | |
CAS No. |
40904-90-3 | |
Record name | 5,10,15,20-tetrapyridin-2-yl-21,22-dihydroporphyrin | |
Source | EPA DSSTox | |
URL | https://comptox.epa.gov/dashboard/DTXSID10408775 | |
Description | DSSTox provides a high quality public chemistry resource for supporting improved predictive toxicology. | |
Retrosynthesis Analysis
AI-Powered Synthesis Planning: Our tool employs the Template_relevance Pistachio, Template_relevance Bkms_metabolic, Template_relevance Pistachio_ringbreaker, Template_relevance Reaxys, Template_relevance Reaxys_biocatalysis model, leveraging a vast database of chemical reactions to predict feasible synthetic routes.
One-Step Synthesis Focus: Specifically designed for one-step synthesis, it provides concise and direct routes for your target compounds, streamlining the synthesis process.
Accurate Predictions: Utilizing the extensive PISTACHIO, BKMS_METABOLIC, PISTACHIO_RINGBREAKER, REAXYS, REAXYS_BIOCATALYSIS database, our tool offers high-accuracy predictions, reflecting the latest in chemical research and data.
Strategy Settings
Precursor scoring | Relevance Heuristic |
---|---|
Min. plausibility | 0.01 |
Model | Template_relevance |
Template Set | Pistachio/Bkms_metabolic/Pistachio_ringbreaker/Reaxys/Reaxys_biocatalysis |
Top-N result to add to graph | 6 |
Feasible Synthetic Routes
Q1: How does the introduction of chlorine atoms at the β-positions of the porphyrin ring influence the electrochemical properties and superoxide dismutase (SOD) activity of Manganese(III) 5,10,15,20-Tetrakis(N-ethylpyridinium-2-yl)porphyrin?
A1: Research indicates that progressive β-chlorination of Manganese(III) 5,10,15,20-Tetrakis(N-ethylpyridinium-2-yl)porphyrin leads to a significant increase in its redox potential, directly correlating with enhanced SOD activity. [] Each chlorine atom added to the porphyrin ring results in an average increase of 55 mV in the redox potential. This increase is attributed to the electron-withdrawing nature of chlorine, which makes the manganese center more electron-deficient and thus a more potent oxidant for superoxide. Consequently, the tetrachlorinated derivative exhibits the highest SOD rate constant (kcat = 4.0 × 10⁸ M⁻¹ s⁻¹) compared to the non-chlorinated analog (kcat = 5.7 × 10⁷ M⁻¹ s⁻¹). [] This observation highlights the impact of structural modifications on the electrochemical properties and catalytic activity of metalloporphyrins.
Q2: What are the key structural features of (trans-dihydroxo)[5,10,15,20-tetrakis(2-pyridyl)porphyrinato]tin(IV) and how are they confirmed?
A2: This tin(IV)-porphyrin complex is characterized by the presence of two trans-dihydroxo axial ligands bound to the central tin atom. [] The 5,10,15,20-tetrakis(2-pyridyl)porphyrin macrocycle provides four nitrogen atoms for coordination, resulting in a six-coordinate tin center. The structural characterization of this complex has been achieved through a combination of techniques, including elemental analysis, ¹H NMR spectroscopy, ESI-MS spectrometry, UV-visible spectroscopy, and fluorescence spectroscopy. [] These methods provide complementary information about the elemental composition, connectivity of atoms, molecular weight, and electronic properties of the complex, confirming its structure and purity.
Haftungsausschluss und Informationen zu In-Vitro-Forschungsprodukten
Bitte beachten Sie, dass alle Artikel und Produktinformationen, die auf BenchChem präsentiert werden, ausschließlich zu Informationszwecken bestimmt sind. Die auf BenchChem zum Kauf angebotenen Produkte sind speziell für In-vitro-Studien konzipiert, die außerhalb lebender Organismen durchgeführt werden. In-vitro-Studien, abgeleitet von dem lateinischen Begriff "in Glas", beinhalten Experimente, die in kontrollierten Laborumgebungen unter Verwendung von Zellen oder Geweben durchgeführt werden. Es ist wichtig zu beachten, dass diese Produkte nicht als Arzneimittel oder Medikamente eingestuft sind und keine Zulassung der FDA für die Vorbeugung, Behandlung oder Heilung von medizinischen Zuständen, Beschwerden oder Krankheiten erhalten haben. Wir müssen betonen, dass jede Form der körperlichen Einführung dieser Produkte in Menschen oder Tiere gesetzlich strikt untersagt ist. Es ist unerlässlich, sich an diese Richtlinien zu halten, um die Einhaltung rechtlicher und ethischer Standards in Forschung und Experiment zu gewährleisten.