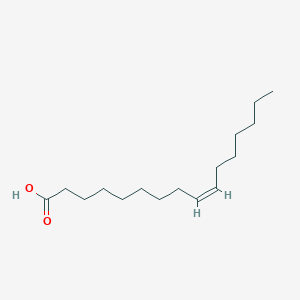
Palmitoleinsäure
Übersicht
Beschreibung
It is a rare component of fats and is commonly found in the glycerides of human adipose tissue . Palmitoleic acid is biosynthesized from palmitic acid by the action of the enzyme Stearoyl-CoA desaturase-1 . It is present in all tissues but is generally found in higher concentrations in the liver . This fatty acid is known for its anti-inflammatory properties and its role in improving insulin sensitivity in liver and skeletal muscles .
Wissenschaftliche Forschungsanwendungen
Palmitoleic acid has a wide range of scientific research applications:
Medicine: It has been studied for its potential in improving insulin sensitivity, reducing inflammation, and promoting cardiovascular health It is also used in wound healing formulations due to its anti-inflammatory properties.
Industry: Palmitoleic acid is used in the cosmetic industry to improve skin hydration and elasticity.
Wirkmechanismus
Target of Action
Palmitoleic acid, a monounsaturated fatty acid, primarily targets adipose tissue and liver cells . It plays a crucial role in the synthesis and breakdown of triglycerides, which are a type of fat stored in adipose tissue and used as a source of energy . It also acts as a signaling molecule, influencing various metabolic processes in the body .
Mode of Action
Palmitoleic acid is synthesized from palmitic acid by the action of the enzyme stearoyl-CoA desaturase-1 . It is known to improve insulin sensitivity in liver and skeletal muscles, and has anti-inflammatory properties . Many of the effects of palmitoleic acid are due to its activation of PPAR-alpha .
Biochemical Pathways
Palmitoleic acid is involved in several biochemical pathways. It is synthesized in the body through the process of fatty acid biosynthesis . It is produced from a precursor fatty acid called palmitic acid, which is a saturated fatty acid with a 16-carbon chain . The conversion of palmitic acid to palmitoleic acid is catalyzed by the enzyme delta-9 desaturase .
Pharmacokinetics
It is known that palmitoleic acid is present in all tissues but is found in higher concentrations in the liver . It is also known that it can be provided in the diet or synthesized endogenously from other fatty acids, carbohydrates, and amino acids .
Result of Action
The action of palmitoleic acid results in several beneficial effects. It has been shown to increase lipolysis, fatty acid oxidation, and ATP content in white adipocytes . It also improves insulin sensitivity, beta cell function, and glucose tolerance . High levels of this fatty acid can also indicate various metabolic imbalances or health concerns .
Action Environment
The action of palmitoleic acid can be influenced by various environmental factors. For example, the presence of other fatty acids, the nutritional status of the individual, and the individual’s energy expenditure can all affect the action of palmitoleic acid . Furthermore, the effects of palmitoleic acid can be influenced by the presence of certain diseases, such as obesity and diabetes .
Biochemische Analyse
Biochemical Properties
Palmitoleic acid is synthesized in the body through the process of fatty acid biosynthesis . It is produced from a precursor fatty acid called palmitic acid, which is a saturated fatty acid with a 16-carbon chain . Experimental studies suggest that palmitoleic acid may act as an adipocyte-derived lipid hormone (or ‘lipokine’) to regulate systemic metabolism .
Cellular Effects
Circulating palmitoleic acid is an independent determinant of insulin sensitivity, beta cell function, and glucose tolerance in non-diabetic individuals . High palmitoleic acid concentrations prevent the decrease in insulin sensitivity associated with excess palmitate .
Molecular Mechanism
Palmitoleic acid exerts its effects at the molecular level by interacting with various biomolecules. It has been shown to suppress hepatic steatosis and improve insulin sensitivity . This suggests that palmitoleic acid may bind to certain biomolecules, inhibit or activate enzymes, and cause changes in gene expression .
Temporal Effects in Laboratory Settings
The effects of palmitoleic acid on cellular function have been observed over time in laboratory settings. For instance, a positive independent relationship was observed between changes in palmitoleic acid and insulin sensitivity over time .
Dosage Effects in Animal Models
The effects of palmitoleic acid vary with different dosages in animal models. While specific dosage effects are not mentioned in the references, it is known that palmitoleic acid has beneficial effects on metabolic disorders .
Metabolic Pathways
Palmitoleic acid is involved in various metabolic pathways. It is produced from palmitic acid through the process of fatty acid biosynthesis . The specific enzymes or cofactors it interacts with are not mentioned in the references.
Transport and Distribution
Given its role in systemic metabolism, it can be inferred that it is likely transported and distributed in a manner that allows it to interact with various cells and tissues .
Subcellular Localization
Given its role in systemic metabolism and its interaction with various biomolecules, it can be inferred that it is likely localized in areas of the cell where these interactions occur .
Vorbereitungsmethoden
Synthetic Routes and Reaction Conditions: Palmitoleic acid can be enriched from natural sources such as sea buckthorn pulp oil through a combination of two-step solvent crystallization and molecular distillation . The process involves converting sea buckthorn pulp oil to its corresponding mixed fatty acids, followed by primary and secondary crystallization using methanol at specific temperatures and ratios . The final purification is achieved through molecular distillation at an optimal temperature of 100°C .
Industrial Production Methods: Industrial production of palmitoleic acid often involves the extraction from natural sources like macadamia oil and sea buckthorn oil, which contain high concentrations of this fatty acid . The extracted oil undergoes processes such as solvent crystallization and molecular distillation to obtain a concentrated form of palmitoleic acid .
Analyse Chemischer Reaktionen
Types of Reactions: Palmitoleic acid undergoes various chemical reactions, including oxidation, reduction, and substitution .
Common Reagents and Conditions:
Substitution: Halogenation reactions can occur with reagents like bromine, leading to the formation of dibromo derivatives.
Major Products Formed:
Oxidation: Epoxides and diols
Reduction: Palmitic acid
Substitution: Dibromo derivatives
Vergleich Mit ähnlichen Verbindungen
Palmitic Acid: A saturated fatty acid with a similar carbon chain length but without the double bond present in palmitoleic acid.
Sapienic Acid: An omega-10 monounsaturated fatty acid with a similar structure but with the double bond located at the tenth carbon from the carboxyl end.
Uniqueness of Palmitoleic Acid: Palmitoleic acid is unique due to its omega-7 configuration, which gives it distinct properties and functions compared to other fatty acids . Its role in improving insulin sensitivity and reducing inflammation sets it apart from other similar compounds .
Eigenschaften
CAS-Nummer |
373-49-9 |
---|---|
Molekularformel |
C16H30O2 |
Molekulargewicht |
254.41 g/mol |
IUPAC-Name |
hexadec-9-enoic acid |
InChI |
InChI=1S/C16H30O2/c1-2-3-4-5-6-7-8-9-10-11-12-13-14-15-16(17)18/h7-8H,2-6,9-15H2,1H3,(H,17,18) |
InChI-Schlüssel |
SECPZKHBENQXJG-UHFFFAOYSA-N |
SMILES |
CCCCCCC=CCCCCCCCC(=O)O |
Isomerische SMILES |
CCCCCC/C=C\CCCCCCCC(=O)O |
Kanonische SMILES |
CCCCCCC=CCCCCCCCC(=O)O |
Aussehen |
Unit:100 mgSolvent:nonePurity:99%Physical liquid |
melting_point |
-0.1 °C |
Key on ui other cas no. |
373-49-9 2091-29-4 |
Physikalische Beschreibung |
Liquid, Other Solid; Liquid |
Piktogramme |
Irritant |
Synonyme |
(9Z)-9-Hexadecenoic Acid; (Z)-9-Hexadecenoic Acid; (Z)-Hexadec-9-enoic Acid; 9-Hexadecenoic Acid; 9-cis-Hexadecenoic Acid; 9Z-Hexadecenoic Acid; C16:1; Oleopalmitic Acid; Zoomeric Acid; cis-9-Hexadecenoic Acid; cis-Palmitoleic Acid; cis-Δ9-Hexadeceno |
Herkunft des Produkts |
United States |
Retrosynthesis Analysis
AI-Powered Synthesis Planning: Our tool employs the Template_relevance Pistachio, Template_relevance Bkms_metabolic, Template_relevance Pistachio_ringbreaker, Template_relevance Reaxys, Template_relevance Reaxys_biocatalysis model, leveraging a vast database of chemical reactions to predict feasible synthetic routes.
One-Step Synthesis Focus: Specifically designed for one-step synthesis, it provides concise and direct routes for your target compounds, streamlining the synthesis process.
Accurate Predictions: Utilizing the extensive PISTACHIO, BKMS_METABOLIC, PISTACHIO_RINGBREAKER, REAXYS, REAXYS_BIOCATALYSIS database, our tool offers high-accuracy predictions, reflecting the latest in chemical research and data.
Strategy Settings
Precursor scoring | Relevance Heuristic |
---|---|
Min. plausibility | 0.01 |
Model | Template_relevance |
Template Set | Pistachio/Bkms_metabolic/Pistachio_ringbreaker/Reaxys/Reaxys_biocatalysis |
Top-N result to add to graph | 6 |
Feasible Synthetic Routes
Haftungsausschluss und Informationen zu In-Vitro-Forschungsprodukten
Bitte beachten Sie, dass alle Artikel und Produktinformationen, die auf BenchChem präsentiert werden, ausschließlich zu Informationszwecken bestimmt sind. Die auf BenchChem zum Kauf angebotenen Produkte sind speziell für In-vitro-Studien konzipiert, die außerhalb lebender Organismen durchgeführt werden. In-vitro-Studien, abgeleitet von dem lateinischen Begriff "in Glas", beinhalten Experimente, die in kontrollierten Laborumgebungen unter Verwendung von Zellen oder Geweben durchgeführt werden. Es ist wichtig zu beachten, dass diese Produkte nicht als Arzneimittel oder Medikamente eingestuft sind und keine Zulassung der FDA für die Vorbeugung, Behandlung oder Heilung von medizinischen Zuständen, Beschwerden oder Krankheiten erhalten haben. Wir müssen betonen, dass jede Form der körperlichen Einführung dieser Produkte in Menschen oder Tiere gesetzlich strikt untersagt ist. Es ist unerlässlich, sich an diese Richtlinien zu halten, um die Einhaltung rechtlicher und ethischer Standards in Forschung und Experiment zu gewährleisten.