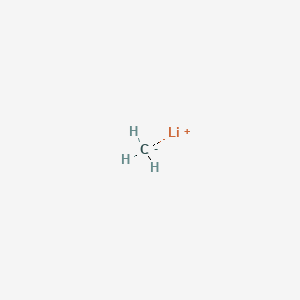
Methyllithium
Übersicht
Beschreibung
Methyllithium (MeLi) is a fundamental organolithium reagent with the chemical formula [Li+].[CH3-], characterized by its high basicity and nucleophilicity . It is commercially available in ethereal solutions (e.g., diethyl ether or diethoxymethane) at concentrations ranging from 1.6 M to 3.1 M . MeLi is widely used in deprotonation reactions, methyl anion transfer, and the synthesis of complex organometallic compounds such as lithium dimethylcuprate . Its reactivity is highly solvent-dependent, with tetrahydrofuran (THF) enhancing its nucleophilicity due to stronger lithium coordination .
Vorbereitungsmethoden
Direct Reaction of Lithium Metal with Methyl Halides
Mechanistic Foundations
The direct reaction between lithium metal and methyl halides (CH₃X, X = Cl, Br, I) remains the most widely employed method for MeLi synthesis. The process involves a single-electron transfer (SET) mechanism, where lithium donates an electron to the carbon-halogen bond, leading to homolytic cleavage and subsequent formation of MeLi and lithium halide (LiX) . Chloromethane (CH₃Cl) is preferred industrially due to its balance of reactivity and cost-effectiveness.
Solvent Systems and Stabilization
Critical to this method is the choice of solvent, which must dissolve lithium, facilitate electron transfer, and stabilize the reactive MeLi product. Aromatic hydrocarbons such as toluene, cumene, and ethylbenzene are commonly used due to their inertness and compatibility with lithium dispersions . Recent advancements highlight the role of methyltetrahydrofuran (MeTHF) as a co-solvent, which enhances stability by coordinating to lithium without inducing excessive aggregation.
Table 1: Solvent Systems in MeLi Synthesis
Solvent Combination | Stability (30 days at 40°C) | Decomposition Rate |
---|---|---|
Toluene/THF (1:2) | 86% | 14% |
Cumene/MeTHF (1:2.5) | 95% | 5% |
Ethylbenzene/MeTHF (1:3) | 94% | 6% |
Lithium Dispersion Techniques
Preparation of Lithium Dispersions
Industrial-scale MeLi production relies on finely dispersed lithium metal to maximize surface area and reaction efficiency. A patented method involves melting lithium (and optionally sodium) in an inert hydrocarbon (e.g., hexane, heptane) at 180–200°C under high-shear agitation (3,450 rpm). The addition of 3–5 wt% sodium accelerates dispersion formation by lowering the melting point and modifying surface morphology .
Role of Dispersing Agents
Linseed oil (0.3–0.65 wt%) or oleic acid is introduced during dispersion to prevent particle coalescence. The resultant lithium-sodium dispersion exhibits a mean particle size of 5–60 μm, optimal for subsequent reaction with chloromethane .
Reaction Engineering and Process Optimization
Temperature and Pressure Control
The exothermic nature of the Li/CH₃Cl reaction necessitates precise temperature control. Batch reactors are typically maintained at −40°C to 50°C, with gaseous chloromethane introduced at 1–5 atmospheres to minimize side reactions . Cooling systems integrated into reactor jackets ensure thermal regulation, while inert gas purging (argon) prevents oxidation.
Solvent Ratios and Equivalents
Stoichiometric ratios of MeTHF to MeLi (2.1–3:1) are critical for stability. Excess MeTHF (>4 equivalents) risks solvolysis, while insufficient amounts (<2 equivalents) lead to premature precipitation of LiCl byproducts .
Table 2: Optimal Reaction Parameters
Parameter | Range |
---|---|
Temperature | −40°C to 50°C |
Pressure (CH₃Cl) | 1–5 atm |
MeTHF:MeLi Ratio | 2.1–3:1 |
Agitation Speed | 1,425–3,450 rpm |
Stabilization via Lewis Base Coordination
MeTHF vs. THF: A Stability Comparison
MeTHF’s superior electron-donating ability and lower basicity compared to THF reduce LiCl co-complexation, yielding solutions with <6% decomposition after 30 days at 40°C. In contrast, THF-based formulations degrade by 14% under identical conditions .
Sodium Additives
Incorporating 3–5 wt% sodium in lithium dispersions enhances reactivity by disrupting the lithium oxide passivation layer. This modification reduces induction periods and improves conversion rates by 20–30% .
Monomeric this compound Complexes
Ligand-Stabilized Synthesis
Recent academic work demonstrates the isolation of monomeric MeLi using polydentate amines. For example, [Li(CH₃)(Me₆Tren)] (Me₆Tren = tris(N,N-dimethylaminoethyl)amine) forms a stable monomeric structure confirmed by single-crystal X-ray diffraction (SCXRD) . This approach prevents aggregation and enables precise control over reactivity, though scalability remains a challenge.
Table 3: Ligand Effects on MeLi Stability
Ligand | Aggregation State | Decomposition Pathway |
---|---|---|
Me₆Tren | Monomeric | C–H Activation |
DETAN | Dimeric | Ligand Degradation |
Unligated (THF) | Hexameric | Solvolysis |
Industrial-Scale Production
Analyse Chemischer Reaktionen
Types of Reactions: Methyllithium undergoes various types of reactions, including nucleophilic addition, deprotonation, and substitution reactions. It is both strongly basic and highly nucleophilic due to the partial negative charge on carbon .
Common Reagents and Conditions:
Nucleophilic Addition: this compound reacts with ketones to give tertiary alcohols in a two-step process: [ \text{Ph}_2\text{CO} + \text{CH}_3\text{Li} \rightarrow \text{Ph}_2\text{C}(\text{CH}_3)\text{OLi} ] [ \text{Ph}_2\text{C}(\text{CH}_3)\text{OLi} + \text{H}^+ \rightarrow \text{Ph}_2\text{C}(\text{CH}_3)\text{OH} + \text{Li}^+ ]
Substitution: Nonmetal halides are converted to methyl compounds with this compound: [ \text{PCl}_3 + 3 \text{CH}_3\text{Li} \rightarrow \text{P(CH}_3)_3 + 3 \text{LiCl} ]
Major Products: The major products formed from these reactions include tertiary alcohols and methylated nonmetal compounds .
Wissenschaftliche Forschungsanwendungen
Key Applications
- Synthesis of Alcohols
- Formation of Organocopper Compounds
- Reactions with Carbon Dioxide
- Synthesis of Methyl Compounds
Case Study 1: Isolation of Chloride-Free this compound
A recent study focused on isolating chloride-free this compound to improve the purity of reactions involving this reagent. The researchers employed K[N(SiMe₃)₂] as a halide-trapping reagent, demonstrating that this approach significantly reduced contamination from lithium halides, which can complicate synthesis protocols .
Case Study 2: Applications in Drug Synthesis
This compound has been utilized in synthesizing complex pharmaceuticals by facilitating the formation of key intermediates. For instance, it was employed to produce allylic alcohols from acrolein with a yield of 50%, showcasing its effectiveness in multi-step organic syntheses .
Data Table: Summary of Reactions Involving this compound
Reaction Type | Reactants | Products | Yield (%) |
---|---|---|---|
Alcohol Synthesis | Ketone + this compound | Tertiary Alcohol | Variable |
Organocopper Formation | Metal Halide + this compound | Organocopper Compound | High |
Carbon Dioxide Reaction | This compound + CO₂ | Lithium Acetate | High |
Methylation of Halides | Nonmetal Halide + this compound | Methyl Compound | Variable |
Wirkmechanismus
Methyllithium exerts its effects through nucleophilic addition and deprotonation reactions. The partial negative charge on the carbon atom makes it highly reactive towards electron acceptors and proton donors. This reactivity is crucial for its role in organic synthesis and other applications .
Vergleich Mit ähnlichen Verbindungen
Comparison with Structurally Similar Organolithium Compounds
n-Butyllithium (n-BuLi)
- Structure and Basicity : n-BuLi has a longer alkyl chain (C₄H₉Li), making it less basic but more nucleophilic than MeLi. Its bulkier structure reduces accessibility to sterically hindered substrates .
- Applications : Primarily used for deprotonation of stronger acids (e.g., alkenes, arenes), whereas MeLi is preferred for methylations and reactions requiring smaller nucleophiles .
- Halide Contamination : Unlike MeLi, n-BuLi is typically synthesized without halide byproducts, avoiding interference in cuprate formation .
Isobutyllithium (i-BuLi)
- Reactivity : The branched structure of i-BuLi increases steric hindrance, reducing its reactivity toward bulky substrates compared to MeLi. For example, in the synthesis of indole intermediates, i-BuLi generates different products due to altered nucleophilic pathways .
- Thermodynamic Stability : i-BuLi forms more stable aggregates in solution, which can slow reaction kinetics relative to MeLi .
[Bis(Trimethylsilyl)Methyl]Lithium
- Solubility : Modified with trimethylsilyl groups, this reagent exhibits exceptional solubility in alkanes, unlike MeLi, which requires polar solvents like ethers .
- Coordination Chemistry: Forms stable complexes with O- and N-donor ligands, a property leveraged in catalysis and materials science. MeLi, in contrast, predominantly forms transient aggregates with solvents like THF .
Functional Comparisons with Lithium Halides and Amides
Lithium Halides (LiCl, LiBr)
- Aggregation Effects: LiCl and LiBr form mixed aggregates with MeLi, displacing chiral lithium amides and reducing enantioselectivity in asymmetric reactions (e.g., 1-o-tolylethanol synthesis) .
- Interference in Reactions : Halide-contaminated MeLi solutions (common in commercial preparations) hinder cuprate synthesis and alter stereochemical outcomes in carbonyl additions .
Lithium Amides (e.g., LDA, LiHMDS)
- Basicity vs. Nucleophilicity : Lithium amides are stronger bases but weaker nucleophiles than MeLi. For instance, lithium diisopropylamide (LDA) deprotonates substrates without transferring methyl groups .
- Chiral Induction : Chiral lithium amides (e.g., 3APLi) are often used with MeLi for asymmetric alkylations, but their efficacy is compromised by lithium halides through competitive aggregation .
Deprotonation and Methyl Transfer
- Substrate Scope: MeLi deprotonates weakly acidic substrates (pKa ~25–35 in THF), such as silanolates and enol ethers, whereas bulkier reagents like n-BuLi target stronger acids (pKa <25) .
- Methylation Efficiency: In reactions with α-lithiated arylacetonitriles, MeLi acts solely as a base, while other organolithiums (e.g., α-lithiated nitriles) participate in nucleophilic substitution .
Asymmetric Catalysis
- Enantioselectivity : MeLi’s small size enables precise stereochemical control in allylic alkylations, but its sensitivity to lithium halides necessitates rigorous purification for high enantiomeric excess .
Data Tables
Table 1: Physical and Chemical Properties
Table 2: Reaction Outcomes with Different Reagents
Biologische Aktivität
Methyllithium (MeLi) is a highly reactive organolithium compound widely used in organic synthesis. Its biological activity, while less studied than its chemical reactivity, is of interest due to its potential applications in medicinal chemistry and its interactions with biological systems. This article reviews the biological activity of this compound, focusing on its mechanisms of action, structural characteristics, and relevant case studies.
This compound is an organolithium reagent characterized by the formula . It exists primarily as a monomer in nonpolar solvents, exhibiting significant nucleophilicity due to the presence of lithium. This property allows it to participate in various reactions, including nucleophilic additions and carbon-carbon bond formations.
Table 1: Key Properties of this compound
Property | Value |
---|---|
Molecular Weight | 23.95 g/mol |
Melting Point | -108 °C |
Boiling Point | -34 °C |
Solubility | Soluble in diethyl ether |
Structure | Monomeric (CH₃Li) |
Mechanisms of Biological Activity
The biological activity of this compound is primarily linked to its interaction with cellular components and enzymes. One significant area of research involves its effect on protein structures and functions through metal coordination and nucleophilic attack.
- Interaction with Proteins : this compound can form complexes with proteins, potentially altering their conformation and activity. Recent studies have shown that this compound can react with amino acid side chains, leading to modifications that may impact enzyme function.
- Inhibition of Enzymatic Activity : this compound has been observed to inhibit certain enzymes by modifying their active sites. For example, it can react with the thiol groups in cysteine residues, which are crucial for the catalytic activity of various enzymes.
- Impact on Cell Signaling : The compound may influence cell signaling pathways by altering the phosphorylation states of proteins involved in these pathways. This can lead to downstream effects on gene expression and cellular responses.
Case Study 1: this compound and Enzyme Inhibition
A study investigated the effects of this compound on a specific enzyme involved in metabolic pathways. The results indicated that this compound inhibited the enzyme's activity significantly by modifying key cysteine residues at the active site, leading to reduced substrate turnover rates.
Case Study 2: Cellular Uptake and Toxicity
Research has demonstrated that this compound can penetrate cellular membranes, where it interacts with intracellular proteins. While this property can be exploited for drug delivery systems, it also raises concerns regarding cytotoxicity due to potential off-target effects.
Structural Studies
Recent advancements in structural analysis techniques have provided insights into the behavior of this compound in biological contexts. For instance, X-ray crystallography has been employed to elucidate the structure of this compound complexes with various ligands, revealing how these interactions may influence biological activity.
Table 2: Structural Characteristics from Recent Studies
Eigenschaften
IUPAC Name |
lithium;carbanide | |
---|---|---|
Source | PubChem | |
URL | https://pubchem.ncbi.nlm.nih.gov | |
Description | Data deposited in or computed by PubChem | |
InChI |
InChI=1S/CH3.Li/h1H3;/q-1;+1 | |
Source | PubChem | |
URL | https://pubchem.ncbi.nlm.nih.gov | |
Description | Data deposited in or computed by PubChem | |
InChI Key |
IHLVCKWPAMTVTG-UHFFFAOYSA-N | |
Source | PubChem | |
URL | https://pubchem.ncbi.nlm.nih.gov | |
Description | Data deposited in or computed by PubChem | |
Canonical SMILES |
[Li+].[CH3-] | |
Source | PubChem | |
URL | https://pubchem.ncbi.nlm.nih.gov | |
Description | Data deposited in or computed by PubChem | |
Molecular Formula |
CH3Li | |
Source | PubChem | |
URL | https://pubchem.ncbi.nlm.nih.gov | |
Description | Data deposited in or computed by PubChem | |
DSSTOX Substance ID |
DTXSID7061273 | |
Record name | Lithium, methyl- | |
Source | EPA DSSTox | |
URL | https://comptox.epa.gov/dashboard/DTXSID7061273 | |
Description | DSSTox provides a high quality public chemistry resource for supporting improved predictive toxicology. | |
Molecular Weight |
22.0 g/mol | |
Source | PubChem | |
URL | https://pubchem.ncbi.nlm.nih.gov | |
Description | Data deposited in or computed by PubChem | |
CAS No. |
917-54-4 | |
Record name | Lithium, methyl- | |
Source | ChemIDplus | |
URL | https://pubchem.ncbi.nlm.nih.gov/substance/?source=chemidplus&sourceid=0000917544 | |
Description | ChemIDplus is a free, web search system that provides access to the structure and nomenclature authority files used for the identification of chemical substances cited in National Library of Medicine (NLM) databases, including the TOXNET system. | |
Record name | Lithium, methyl- | |
Source | EPA Chemicals under the TSCA | |
URL | https://www.epa.gov/chemicals-under-tsca | |
Description | EPA Chemicals under the Toxic Substances Control Act (TSCA) collection contains information on chemicals and their regulations under TSCA, including non-confidential content from the TSCA Chemical Substance Inventory and Chemical Data Reporting. | |
Record name | Lithium, methyl- | |
Source | EPA DSSTox | |
URL | https://comptox.epa.gov/dashboard/DTXSID7061273 | |
Description | DSSTox provides a high quality public chemistry resource for supporting improved predictive toxicology. | |
Record name | Methyllithium | |
Source | European Chemicals Agency (ECHA) | |
URL | https://echa.europa.eu/substance-information/-/substanceinfo/100.011.843 | |
Description | The European Chemicals Agency (ECHA) is an agency of the European Union which is the driving force among regulatory authorities in implementing the EU's groundbreaking chemicals legislation for the benefit of human health and the environment as well as for innovation and competitiveness. | |
Explanation | Use of the information, documents and data from the ECHA website is subject to the terms and conditions of this Legal Notice, and subject to other binding limitations provided for under applicable law, the information, documents and data made available on the ECHA website may be reproduced, distributed and/or used, totally or in part, for non-commercial purposes provided that ECHA is acknowledged as the source: "Source: European Chemicals Agency, http://echa.europa.eu/". Such acknowledgement must be included in each copy of the material. ECHA permits and encourages organisations and individuals to create links to the ECHA website under the following cumulative conditions: Links can only be made to webpages that provide a link to the Legal Notice page. | |
Haftungsausschluss und Informationen zu In-Vitro-Forschungsprodukten
Bitte beachten Sie, dass alle Artikel und Produktinformationen, die auf BenchChem präsentiert werden, ausschließlich zu Informationszwecken bestimmt sind. Die auf BenchChem zum Kauf angebotenen Produkte sind speziell für In-vitro-Studien konzipiert, die außerhalb lebender Organismen durchgeführt werden. In-vitro-Studien, abgeleitet von dem lateinischen Begriff "in Glas", beinhalten Experimente, die in kontrollierten Laborumgebungen unter Verwendung von Zellen oder Geweben durchgeführt werden. Es ist wichtig zu beachten, dass diese Produkte nicht als Arzneimittel oder Medikamente eingestuft sind und keine Zulassung der FDA für die Vorbeugung, Behandlung oder Heilung von medizinischen Zuständen, Beschwerden oder Krankheiten erhalten haben. Wir müssen betonen, dass jede Form der körperlichen Einführung dieser Produkte in Menschen oder Tiere gesetzlich strikt untersagt ist. Es ist unerlässlich, sich an diese Richtlinien zu halten, um die Einhaltung rechtlicher und ethischer Standards in Forschung und Experiment zu gewährleisten.