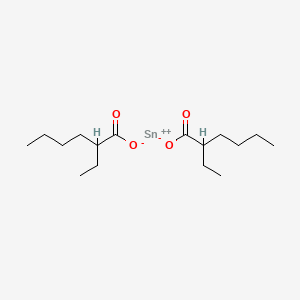
Zinn(II)-octoat
Übersicht
Beschreibung
It is a clear, colorless liquid at room temperature, although it often appears yellow due to impurities resulting from the oxidation of tin(II) to tin(IV) . This compound is widely used as a catalyst in various chemical reactions, particularly in the polymer industry for the production of polylactic acid and other polymers .
Wissenschaftliche Forschungsanwendungen
Stannous octoate is extensively used in scientific research and industrial applications, including:
Polymerization: It is a highly effective catalyst for the ring-opening polymerization of lactides and other cyclic esters, leading to the production of biodegradable polymers such as polylactic acid
Biomedical Applications: Polylactic acid produced using stannous octoate is used in medical devices, drug delivery systems, and tissue engineering scaffolds.
Wirkmechanismus
Target of Action
Stannous octoate, also known as tin(II) 2-ethylhexanoate or tin(II) octoate, is primarily used as a catalyst in the synthesis of polymeric materials . Its primary targets are the monomers of these polymers, such as D,L-lactide and epsilon-caprolactone . These monomers are involved in the production of elastomeric copolymers and polylactic acid .
Mode of Action
Stannous octoate interacts with its targets through a process known as ring-opening polymerization . In this process, the stannous octoate acts as a catalyst, facilitating the reaction between the monomers to form long-chain polymers . The results were consistent with a mechanism in which stannous alkoxide initiator is formed in situ via reaction between stannous octoate and alcohol, and stannous alkoxide chain ends are the actively propagating species .
Biochemical Pathways
The primary biochemical pathway affected by stannous octoate is the polymerization of lactides and other cyclic esters . This process results in the formation of polymeric materials, such as elastomeric copolymers and polylactic acid . These materials have a wide range of applications, including the production of flexible foams for mattresses, furniture, and carpeting markets .
Pharmacokinetics
It is known that stannous octoate is a clear colorless liquid at room temperature, though often appears yellow due to impurities, likely resulting from oxidation of sn(ii) to sn(iv) .
Result of Action
The result of stannous octoate’s action is the formation of polymeric materials with tailored mechanical properties and controlled degradation rates . For example, when combined with hydroxyapatite, the resulting copolymers can create bone-filling composites . These materials have significant potential for use in medical applications, such as tissue engineering and drug delivery .
Action Environment
The action of stannous octoate can be influenced by various environmental factors. For instance, it is known to degrade in water to form Sn(IV) . Therefore, the presence of water can influence the efficacy and stability of stannous octoate. Additionally, it should be stored in a cool, ventilated area away from heat sources and sunlight to ensure safety .
Biochemische Analyse
Biochemical Properties
Stannous octoate plays a significant role in biochemical reactions, primarily as a catalyst. It interacts with various enzymes and proteins, facilitating the polymerization process. For instance, stannous octoate is known to catalyze the ring-opening polymerization of lactides, which is a critical step in the synthesis of polylactic acid . The compound interacts with the hydroxyl groups of the monomers, initiating the polymerization process. Additionally, stannous octoate has been observed to interact with hydroxyapatite, forming composites with tailored mechanical properties and controlled degradation rates .
Cellular Effects
Stannous octoate influences various cellular processes, including cell signaling pathways, gene expression, and cellular metabolism. In particular, it has been shown to affect oxidative phosphorylation, a crucial metabolic pathway for energy production in cells . By inhibiting enzymes involved in this pathway, stannous octoate can lead to cellular dysfunction and adverse health effects. Furthermore, stannous octoate has been reported to cause skin and eye irritation, respiratory issues, and reproductive toxicity at high doses .
Molecular Mechanism
The molecular mechanism of stannous octoate involves its interaction with biomolecules, leading to enzyme inhibition or activation and changes in gene expression. Stannous octoate acts as a catalyst by binding to the active sites of enzymes, facilitating the polymerization of monomers . This binding interaction is crucial for the initiation and propagation of the polymerization process. Additionally, stannous octoate has been shown to inhibit enzymes involved in oxidative phosphorylation, disrupting cellular energy production .
Temporal Effects in Laboratory Settings
In laboratory settings, the effects of stannous octoate can change over time due to its stability and degradation. Stannous octoate is known to degrade in water, forming tin(IV) compounds . This degradation can affect its catalytic activity and long-term effects on cellular function. Studies have shown that stannous octoate can degrade within Ringer solutions over a period of six weeks, with the incorporation of hydroxyapatite reducing the degradation rate .
Dosage Effects in Animal Models
The effects of stannous octoate vary with different dosages in animal models. At low doses, stannous octoate acts as an effective catalyst without causing significant adverse effects. At high doses, it can lead to toxicity, including skin and eye irritation, respiratory issues, and reproductive toxicity . Studies have shown that the rate of polymerization increases with higher concentrations of stannous octoate, but this also raises the risk of adverse effects .
Metabolic Pathways
Stannous octoate is involved in various metabolic pathways, particularly those related to polymerization reactions. It interacts with enzymes and cofactors, facilitating the ring-opening polymerization of lactides and other cyclic esters . Additionally, stannous octoate has been reported to inhibit enzymes involved in oxidative phosphorylation, affecting cellular energy production .
Transport and Distribution
Within cells and tissues, stannous octoate is transported and distributed through interactions with transporters and binding proteins. It has been observed to localize in specific cellular compartments, affecting its catalytic activity and function . The distribution of stannous octoate can influence its effectiveness as a catalyst and its potential toxicity.
Subcellular Localization
Stannous octoate’s subcellular localization is crucial for its activity and function. It has been shown to localize in specific cellular compartments, such as the mitochondria, where it can inhibit enzymes involved in oxidative phosphorylation . This localization is essential for its role as a catalyst and its impact on cellular metabolism.
Vorbereitungsmethoden
Stannous octoate is typically synthesized by reacting tin(II) oxide with 2-ethylhexanoic acid. The reaction is carried out in a reaction kettle, where stannous oxide and 2-ethylhexanoic acid are mixed in proportion. The mixture is then stirred and subjected to a vacuum pump to carry out a dehydration reaction at a temperature of 115-130°C until no fraction is obtained. The reaction is continued for 2-3 hours, followed by cooling and filtering to obtain the final product .
In industrial production, a sodium hydroxide solution is first added to react with isooctanoic acid. Then, a rhodium-doped supported antioxidant is added to react with stannous chloride in a nitrogen atmosphere. This method improves the quality and stability of the product .
Analyse Chemischer Reaktionen
Stannous octoate undergoes various chemical reactions, including:
Oxidation: Stannous octoate can be oxidized to form tin(IV) compounds.
Reduction: It can act as a reducing agent in certain reactions.
Substitution: It can participate in substitution reactions where the octoate group is replaced by other ligands.
Common reagents and conditions used in these reactions include alcohols, which can act as chain transfer agents in polymerization reactions . Major products formed from these reactions include polylactic acid and other polymers .
Vergleich Mit ähnlichen Verbindungen
Stannous octoate is often compared with other tin(II) compounds such as tin(II) n-butoxide, tin(II) n-hexoxide, and tin(II) n-octoxide. These compounds also serve as catalysts in polymerization reactions, but stannous octoate is particularly noted for its efficiency and effectiveness in producing high molecular weight polymers . Similar compounds include:
- Tin(II) n-butoxide
- Tin(II) n-hexoxide
- Tin(II) n-octoxide
Stannous octoate stands out due to its ability to produce polymers with desirable properties and its widespread use in industrial applications .
Biologische Aktivität
Stannous octoate, also known as tin(II) 2-ethylhexanoate, is a tin-based compound widely recognized for its catalytic properties in various biochemical and industrial processes. This article explores the biological activity of stannous octoate, focusing on its mechanisms, effects on cellular processes, and implications in biomedical applications.
Chemical Structure and Properties
Stannous octoate is a clear, colorless liquid that can appear yellow due to impurities. It is primarily used as a catalyst in the synthesis of polymeric materials, particularly in the ring-opening polymerization (ROP) of lactides and other cyclic esters . The compound acts by coordinating with monomers and facilitating their polymerization through a series of chemical reactions.
Mechanism of Action
The mechanism involves the formation of stannous alkoxide initiators through the reaction of stannous octoate with alcohols. These initiators then catalyze the ROP process, leading to the production of biodegradable polymers such as polylactic acid (PLA) . The general reaction can be summarized as follows:
This reaction highlights how stannous octoate serves both as a catalyst and a reactant in polymer synthesis.
Biological Effects
Stannous octoate influences various biological processes, particularly through its interactions with enzymes and cellular structures. Here are some key findings regarding its biological activity:
- Cellular Metabolism : Stannous octoate has been shown to affect oxidative phosphorylation, a critical metabolic pathway for ATP production in cells. This interaction may lead to altered energy metabolism depending on the concentration used.
- Gene Expression : The compound can influence gene expression patterns by modulating signaling pathways within cells. This effect is particularly relevant in studies involving cell proliferation and differentiation .
- Toxicity : While stannous octoate acts effectively as a catalyst at low doses, higher concentrations can induce toxicity. Observed adverse effects include skin irritation, respiratory issues, and potential reproductive toxicity .
Table 1: Summary of Biological Effects of Stannous Octoate
Effect | Observation | Reference |
---|---|---|
Cellular Metabolism | Alters oxidative phosphorylation | |
Gene Expression | Modulates signaling pathways | |
Toxicity | Skin irritation, respiratory issues at high doses |
Case Study: Polymerization in Biomedical Applications
In a study examining the use of stannous octoate in the production of PLA for medical applications, researchers found that the compound effectively catalyzed the polymerization process while maintaining biocompatibility. PLA produced using stannous octoate was evaluated for use in drug delivery systems and tissue engineering scaffolds due to its favorable mechanical properties and degradation rates .
Eigenschaften
IUPAC Name |
2-ethylhexanoate;tin(2+) | |
---|---|---|
Source | PubChem | |
URL | https://pubchem.ncbi.nlm.nih.gov | |
Description | Data deposited in or computed by PubChem | |
InChI |
InChI=1S/2C8H16O2.Sn/c2*1-3-5-6-7(4-2)8(9)10;/h2*7H,3-6H2,1-2H3,(H,9,10);/q;;+2/p-2 | |
Source | PubChem | |
URL | https://pubchem.ncbi.nlm.nih.gov | |
Description | Data deposited in or computed by PubChem | |
InChI Key |
KSBAEPSJVUENNK-UHFFFAOYSA-L | |
Source | PubChem | |
URL | https://pubchem.ncbi.nlm.nih.gov | |
Description | Data deposited in or computed by PubChem | |
Canonical SMILES |
CCCCC(CC)C(=O)[O-].CCCCC(CC)C(=O)[O-].[Sn+2] | |
Source | PubChem | |
URL | https://pubchem.ncbi.nlm.nih.gov | |
Description | Data deposited in or computed by PubChem | |
Molecular Formula |
C16H30O4Sn | |
Source | PubChem | |
URL | https://pubchem.ncbi.nlm.nih.gov | |
Description | Data deposited in or computed by PubChem | |
DSSTOX Substance ID |
DTXSID1027138 | |
Record name | Hexanoic acid, 2-ethyl-, tin(2+) salt | |
Source | EPA DSSTox | |
URL | https://comptox.epa.gov/dashboard/DTXSID1027138 | |
Description | DSSTox provides a high quality public chemistry resource for supporting improved predictive toxicology. | |
Molecular Weight |
405.1 g/mol | |
Source | PubChem | |
URL | https://pubchem.ncbi.nlm.nih.gov | |
Description | Data deposited in or computed by PubChem | |
Physical Description |
Liquid; [Sigma-Aldrich MSDS] | |
Record name | Tin(II) 2-ethylhexanoate | |
Source | Haz-Map, Information on Hazardous Chemicals and Occupational Diseases | |
URL | https://haz-map.com/Agents/17714 | |
Description | Haz-Map® is an occupational health database designed for health and safety professionals and for consumers seeking information about the adverse effects of workplace exposures to chemical and biological agents. | |
Explanation | Copyright (c) 2022 Haz-Map(R). All rights reserved. Unless otherwise indicated, all materials from Haz-Map are copyrighted by Haz-Map(R). No part of these materials, either text or image may be used for any purpose other than for personal use. Therefore, reproduction, modification, storage in a retrieval system or retransmission, in any form or by any means, electronic, mechanical or otherwise, for reasons other than personal use, is strictly prohibited without prior written permission. | |
Vapor Pressure |
0.00000001 [mmHg] | |
Record name | Tin(II) 2-ethylhexanoate | |
Source | Haz-Map, Information on Hazardous Chemicals and Occupational Diseases | |
URL | https://haz-map.com/Agents/17714 | |
Description | Haz-Map® is an occupational health database designed for health and safety professionals and for consumers seeking information about the adverse effects of workplace exposures to chemical and biological agents. | |
Explanation | Copyright (c) 2022 Haz-Map(R). All rights reserved. Unless otherwise indicated, all materials from Haz-Map are copyrighted by Haz-Map(R). No part of these materials, either text or image may be used for any purpose other than for personal use. Therefore, reproduction, modification, storage in a retrieval system or retransmission, in any form or by any means, electronic, mechanical or otherwise, for reasons other than personal use, is strictly prohibited without prior written permission. | |
CAS No. |
301-10-0 | |
Record name | Stannous 2-ethylhexanoate | |
Source | ChemIDplus | |
URL | https://pubchem.ncbi.nlm.nih.gov/substance/?source=chemidplus&sourceid=0000301100 | |
Description | ChemIDplus is a free, web search system that provides access to the structure and nomenclature authority files used for the identification of chemical substances cited in National Library of Medicine (NLM) databases, including the TOXNET system. | |
Record name | Hexanoic acid, 2-ethyl-, tin(2+) salt | |
Source | EPA DSSTox | |
URL | https://comptox.epa.gov/dashboard/DTXSID1027138 | |
Description | DSSTox provides a high quality public chemistry resource for supporting improved predictive toxicology. | |
Record name | STANNOUS 2-ETHYLHEXANOATE | |
Source | FDA Global Substance Registration System (GSRS) | |
URL | https://gsrs.ncats.nih.gov/ginas/app/beta/substances/519A78R12Y | |
Description | The FDA Global Substance Registration System (GSRS) enables the efficient and accurate exchange of information on what substances are in regulated products. Instead of relying on names, which vary across regulatory domains, countries, and regions, the GSRS knowledge base makes it possible for substances to be defined by standardized, scientific descriptions. | |
Explanation | Unless otherwise noted, the contents of the FDA website (www.fda.gov), both text and graphics, are not copyrighted. They are in the public domain and may be republished, reprinted and otherwise used freely by anyone without the need to obtain permission from FDA. Credit to the U.S. Food and Drug Administration as the source is appreciated but not required. | |
Retrosynthesis Analysis
AI-Powered Synthesis Planning: Our tool employs the Template_relevance Pistachio, Template_relevance Bkms_metabolic, Template_relevance Pistachio_ringbreaker, Template_relevance Reaxys, Template_relevance Reaxys_biocatalysis model, leveraging a vast database of chemical reactions to predict feasible synthetic routes.
One-Step Synthesis Focus: Specifically designed for one-step synthesis, it provides concise and direct routes for your target compounds, streamlining the synthesis process.
Accurate Predictions: Utilizing the extensive PISTACHIO, BKMS_METABOLIC, PISTACHIO_RINGBREAKER, REAXYS, REAXYS_BIOCATALYSIS database, our tool offers high-accuracy predictions, reflecting the latest in chemical research and data.
Strategy Settings
Precursor scoring | Relevance Heuristic |
---|---|
Min. plausibility | 0.01 |
Model | Template_relevance |
Template Set | Pistachio/Bkms_metabolic/Pistachio_ringbreaker/Reaxys/Reaxys_biocatalysis |
Top-N result to add to graph | 6 |
Feasible Synthetic Routes
Haftungsausschluss und Informationen zu In-Vitro-Forschungsprodukten
Bitte beachten Sie, dass alle Artikel und Produktinformationen, die auf BenchChem präsentiert werden, ausschließlich zu Informationszwecken bestimmt sind. Die auf BenchChem zum Kauf angebotenen Produkte sind speziell für In-vitro-Studien konzipiert, die außerhalb lebender Organismen durchgeführt werden. In-vitro-Studien, abgeleitet von dem lateinischen Begriff "in Glas", beinhalten Experimente, die in kontrollierten Laborumgebungen unter Verwendung von Zellen oder Geweben durchgeführt werden. Es ist wichtig zu beachten, dass diese Produkte nicht als Arzneimittel oder Medikamente eingestuft sind und keine Zulassung der FDA für die Vorbeugung, Behandlung oder Heilung von medizinischen Zuständen, Beschwerden oder Krankheiten erhalten haben. Wir müssen betonen, dass jede Form der körperlichen Einführung dieser Produkte in Menschen oder Tiere gesetzlich strikt untersagt ist. Es ist unerlässlich, sich an diese Richtlinien zu halten, um die Einhaltung rechtlicher und ethischer Standards in Forschung und Experiment zu gewährleisten.