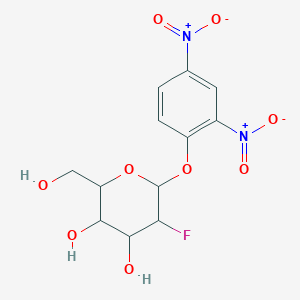
2,4-Dinitrophenyl 2-Deoxy-2-Fluoro-Beta-D-Glucopyranoside
Übersicht
Beschreibung
“2,4-Dinitrophenyl 2-Deoxy-2-Fluoro-Beta-D-Glucopyranoside” belongs to the class of organic compounds known as O-glycosyl compounds. These are glycosides in which a sugar group is bonded through one carbon to another group via an O-glycosidic bond .
Synthesis Analysis
The synthesis of “2,4-Dinitrophenyl 2-Deoxy-2-Fluoro-Beta-D-Glucopyranoside” has been reported in several studies. For instance, the compound was used to crystallize a 50 kDa BGlu1 enzyme by microbatch and hanging-drop vapor diffusion . Another study reported that the compound preferentially inactivates the phlorizin hydrolase activity when treated with LPH .
Molecular Structure Analysis
The molecular formula of “2,4-Dinitrophenyl 2-Deoxy-2-Fluoro-Beta-D-Glucopyranoside” is C12H13FN2O9. The compound has a molecular weight of 348.24 g/mol . The structure of the compound includes a sugar group bonded through one carbon to another group via an O-glycosidic bond .
Chemical Reactions Analysis
“2,4-Dinitrophenyl 2-Deoxy-2-Fluoro-Beta-D-Glucopyranoside” is known to be a mechanism-based inhibitor of glycosidases. When treated with LPH, it preferentially inactivates the phlorizin hydrolase activity .
Physical And Chemical Properties Analysis
The physical and chemical properties of “2,4-Dinitrophenyl 2-Deoxy-2-Fluoro-Beta-D-Glucopyranoside” include a molecular weight of 348.24 g/mol and a molecular formula of C12H13FN2O9 .
Wissenschaftliche Forschungsanwendungen
Biomass Conversion
The compound is used as a mechanism-based inhibitor in the study of endo-xyloglucanases , enzymes that play a crucial role in the metabolism of xyloglucan, a polysaccharide found in plant cell walls . This application is significant for understanding and improving biomass conversion processes, which are essential for producing sustainable fuels and chemicals from plant materials.
Enzyme Mechanism Studies
2,4-Dinitrophenyl 2-Deoxy-2-Fluoro-Beta-D-Glucopyranoside: serves as a tool for structure-function analyses of glycoside hydrolases . By labeling the active sites of these enzymes, researchers can gain insights into the catalytic mechanisms and design more efficient enzymes for industrial applications.
Glycosidase Inhibition
This compound acts as a glycosidase inhibitor, specifically targeting the catalytic nucleophile of anomeric-configuration-retaining glycosidases . This is particularly useful in the development of therapeutic agents against diseases where glycosidases are implicated.
Structural Biology
The inhibitor is used in crystallography to study the covalent nature of enzyme-inhibitor complexes . This helps in elucidating the three-dimensional structures of enzymes, which is fundamental for drug design and other biotechnological applications.
Carbohydrate Chemistry
In the field of carbohydrate chemistry, the synthesis of this compound involves key steps like selective enzyme hydrolysis and electrophilic fluorination . These techniques contribute to the advancement of synthetic methodologies for complex carbohydrates.
Labeling and Tracing
As a labeling agent, 2,4-Dinitrophenyl 2-Deoxy-2-Fluoro-Beta-D-Glucopyranoside can be used to trace the interaction and pathways of carbohydrates in biological systems . This has implications for understanding metabolic processes and the role of carbohydrates in health and disease.
Diagnostic Imaging
Although not directly related to the compound , a similar compound, 2-Deoxy-2-(fluoro-18F)-D-glucose , is used in PET imaging for tracing glucose metabolism in tumors, heart, and brain . This showcases the potential of fluorinated glucose analogs in medical diagnostics.
Chemical Biology
The compound’s ability to specifically label enzyme active sites makes it a valuable tool in chemical biology for studying enzyme-substrate interactions . This can lead to the discovery of new biological pathways and molecular targets for drug development.
Wirkmechanismus
Target of Action
The primary target of 2,4-Dinitrophenyl 2-Deoxy-2-Fluoro-Beta-D-Glucopyranoside is Beta-mannosidase , an enzyme found in the bacterium Bacteroides thetaiotaomicron . This enzyme plays a crucial role in the breakdown of complex carbohydrates.
Mode of Action
The compound interacts with its target, Beta-mannosidase, through a O-glycosidic bond . This bond is formed between a sugar group and another group (in this case, the enzyme) through one carbon
Zukünftige Richtungen
Eigenschaften
IUPAC Name |
(2R,3S,4S,5R,6S)-6-(2,4-dinitrophenoxy)-5-fluoro-2-(hydroxymethyl)oxane-3,4-diol | |
---|---|---|
Source | PubChem | |
URL | https://pubchem.ncbi.nlm.nih.gov | |
Description | Data deposited in or computed by PubChem | |
InChI |
InChI=1S/C12H13FN2O9/c13-9-11(18)10(17)8(4-16)24-12(9)23-7-2-1-5(14(19)20)3-6(7)15(21)22/h1-3,8-12,16-18H,4H2/t8-,9-,10-,11-,12-/m1/s1 | |
Source | PubChem | |
URL | https://pubchem.ncbi.nlm.nih.gov | |
Description | Data deposited in or computed by PubChem | |
InChI Key |
UFSBFVZQJZMIOU-LZQZFOIKSA-N | |
Source | PubChem | |
URL | https://pubchem.ncbi.nlm.nih.gov | |
Description | Data deposited in or computed by PubChem | |
Canonical SMILES |
C1=CC(=C(C=C1[N+](=O)[O-])[N+](=O)[O-])OC2C(C(C(C(O2)CO)O)O)F | |
Source | PubChem | |
URL | https://pubchem.ncbi.nlm.nih.gov | |
Description | Data deposited in or computed by PubChem | |
Isomeric SMILES |
C1=CC(=C(C=C1[N+](=O)[O-])[N+](=O)[O-])O[C@H]2[C@@H]([C@H]([C@@H]([C@H](O2)CO)O)O)F | |
Source | PubChem | |
URL | https://pubchem.ncbi.nlm.nih.gov | |
Description | Data deposited in or computed by PubChem | |
Molecular Formula |
C12H13FN2O9 | |
Source | PubChem | |
URL | https://pubchem.ncbi.nlm.nih.gov | |
Description | Data deposited in or computed by PubChem | |
Molecular Weight |
348.24 g/mol | |
Source | PubChem | |
URL | https://pubchem.ncbi.nlm.nih.gov | |
Description | Data deposited in or computed by PubChem | |
Product Name |
2,4-Dinitrophenyl 2-Deoxy-2-Fluoro-Beta-D-Glucopyranoside |
Retrosynthesis Analysis
AI-Powered Synthesis Planning: Our tool employs the Template_relevance Pistachio, Template_relevance Bkms_metabolic, Template_relevance Pistachio_ringbreaker, Template_relevance Reaxys, Template_relevance Reaxys_biocatalysis model, leveraging a vast database of chemical reactions to predict feasible synthetic routes.
One-Step Synthesis Focus: Specifically designed for one-step synthesis, it provides concise and direct routes for your target compounds, streamlining the synthesis process.
Accurate Predictions: Utilizing the extensive PISTACHIO, BKMS_METABOLIC, PISTACHIO_RINGBREAKER, REAXYS, REAXYS_BIOCATALYSIS database, our tool offers high-accuracy predictions, reflecting the latest in chemical research and data.
Strategy Settings
Precursor scoring | Relevance Heuristic |
---|---|
Min. plausibility | 0.01 |
Model | Template_relevance |
Template Set | Pistachio/Bkms_metabolic/Pistachio_ringbreaker/Reaxys/Reaxys_biocatalysis |
Top-N result to add to graph | 6 |
Feasible Synthetic Routes
Q & A
Q1: How does 2,4-Dinitrophenyl 2-Deoxy-2-Fluoro-Beta-D-Glucopyranoside interact with beta-glucosidases?
A: 2,4-Dinitrophenyl 2-Deoxy-2-Fluoro-Beta-D-Glucopyranoside acts as an inhibitor of beta-glucosidases. While the provided abstracts do not delve into the specific mechanism, it's likely a competitive inhibitor. This means it competes with the natural substrate for binding to the enzyme's active site. The fluorine atom at the 2-position plays a crucial role. This modification is known to alter the substrate's reactivity, potentially slowing down or preventing hydrolysis by the enzyme [, ].
Q2: Why is 2,4-Dinitrophenyl 2-Deoxy-2-Fluoro-Beta-D-Glucopyranoside used in X-ray crystallography studies of beta-glucosidases?
A: This compound is valuable in determining the three-dimensional structure of beta-glucosidases using X-ray crystallography. Researchers formed crystals of both rice BGlu1 [] and Os4BGlu12 [] beta-glucosidases complexed with 2,4-Dinitrophenyl 2-Deoxy-2-Fluoro-Beta-D-Glucopyranoside. The compound, bound to the active site, allows scientists to visualize the enzyme-inhibitor complex at a high resolution. This provides invaluable insights into the enzyme's structure, its interaction with the inhibitor, and potentially helps in designing more potent and specific inhibitors in the future.
Haftungsausschluss und Informationen zu In-Vitro-Forschungsprodukten
Bitte beachten Sie, dass alle Artikel und Produktinformationen, die auf BenchChem präsentiert werden, ausschließlich zu Informationszwecken bestimmt sind. Die auf BenchChem zum Kauf angebotenen Produkte sind speziell für In-vitro-Studien konzipiert, die außerhalb lebender Organismen durchgeführt werden. In-vitro-Studien, abgeleitet von dem lateinischen Begriff "in Glas", beinhalten Experimente, die in kontrollierten Laborumgebungen unter Verwendung von Zellen oder Geweben durchgeführt werden. Es ist wichtig zu beachten, dass diese Produkte nicht als Arzneimittel oder Medikamente eingestuft sind und keine Zulassung der FDA für die Vorbeugung, Behandlung oder Heilung von medizinischen Zuständen, Beschwerden oder Krankheiten erhalten haben. Wir müssen betonen, dass jede Form der körperlichen Einführung dieser Produkte in Menschen oder Tiere gesetzlich strikt untersagt ist. Es ist unerlässlich, sich an diese Richtlinien zu halten, um die Einhaltung rechtlicher und ethischer Standards in Forschung und Experiment zu gewährleisten.