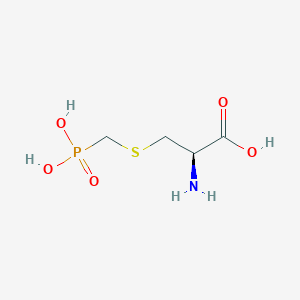
S-Methyl Phosphocysteine
- Klicken Sie auf QUICK INQUIRY, um ein Angebot von unserem Expertenteam zu erhalten.
- Mit qualitativ hochwertigen Produkten zu einem WETTBEWERBSFÄHIGEN Preis können Sie sich mehr auf Ihre Forschung konzentrieren.
Übersicht
Beschreibung
S-Methyl Phosphocysteine is an organic compound belonging to the class of L-cysteine-S-conjugates. It is characterized by the presence of a phosphonomethyl group attached to the sulfur atom of cysteine.
Vorbereitungsmethoden
Synthetische Wege und Reaktionsbedingungen: Die Synthese von S-Methyl-Phosphocystein beinhaltet typischerweise die Konjugation einer Phosphonomethylgruppe an das Schwefelatom von Cystein. Eine gängige Methode beinhaltet die β-Eliminierung von Alkylthiolat aus Cysteinpositionen, gefolgt von der konjugierten Addition von Thiophosphorsäure zu den gebildeten Dehydroalaninresten .
Industrielle Produktionsmethoden: Obwohl spezifische industrielle Produktionsmethoden für S-Methyl-Phosphocystein nicht umfassend dokumentiert sind, beinhaltet der allgemeine Ansatz die großtechnische Synthese unter Verwendung ähnlicher Reaktionsbedingungen wie im Labormaßstab. Die Optimierung von Reaktionsparametern wie Temperatur, pH-Wert und Reagenzkonzentrationen ist für eine effiziente Produktion entscheidend .
Analyse Chemischer Reaktionen
Arten von Reaktionen: S-Methyl-Phosphocystein durchläuft verschiedene chemische Reaktionen, darunter:
Oxidation: Die Verbindung kann oxidiert werden, um Sulfoxide und Sulfone zu bilden.
Reduktion: Reduktionsreaktionen können Sulfoxide wieder zu Sulfiden umwandeln.
Substitution: Nucleophile Substitutionsreaktionen können an der Phosphonomethylgruppe auftreten.
Häufige Reagenzien und Bedingungen:
Oxidation: Häufige Oxidationsmittel sind Wasserstoffperoxid und Persäuren.
Reduktion: Reduktionsmittel wie Natriumborhydrid und Lithiumaluminiumhydrid werden verwendet.
Substitution: Nucleophile wie Amine und Thiole können an Substitutionsreaktionen teilnehmen.
Hauptprodukte:
Oxidation: Sulfoxide und Sulfone.
Reduktion: Sulfide.
Substitution: Verschiedene substituierte Derivate, abhängig vom verwendeten Nucleophil.
Wissenschaftliche Forschungsanwendungen
S-Methyl-Phosphocystein hat vielfältige Anwendungen in der wissenschaftlichen Forschung:
Chemie: Es wird als Baustein in der Synthese komplexer Moleküle und als Ligand in der asymmetrischen Katalyse verwendet.
Biologie: Die Verbindung ist an Signalprozessen und Proteinmodifikationen beteiligt, insbesondere bei der Untersuchung von Proteintyrosinphosphatasen.
Medizin: Die Forschung untersucht sein Potenzial als Therapeutikum, insbesondere bei der Entwicklung von antiviralen Medikamenten.
Industrie: Es wird zur reversiblen Immobilisierung von Aminosäuren oder Peptidderivaten auf Kohlenstoffnanomaterialien verwendet
5. Wirkmechanismus
Der Wirkmechanismus von S-Methyl-Phosphocystein beinhaltet seine Wechselwirkung mit spezifischen molekularen Zielstrukturen und -wegen. Es ist bekannt, dass es mit dem Chemotaxisprotein CheY in Escherichia coli interagiert und die bakterielle Bewegung beeinflusst. Die Phosphonomethylgruppe der Verbindung spielt eine entscheidende Rolle bei ihrer Bindung und Aktivität .
Ähnliche Verbindungen:
S-Phosphocystein: Ähnlich in der Struktur, aber es fehlt die Methylgruppe.
Phosphinothricin: Enthält eine Phosphinatgruppe anstelle einer Phosphonomethylgruppe.
2-Amino-3-Phosphonopropionsäure: Ein Antagonist des metabotropen Glutamatrezeptors.
Einzigartigkeit: S-Methyl-Phosphocystein ist aufgrund seiner spezifischen Phosphonomethylgruppe einzigartig, die im Vergleich zu anderen ähnlichen Verbindungen eine einzigartige chemische Reaktivität und biologische Aktivität verleiht .
Wirkmechanismus
The mechanism of action of S-Methyl Phosphocysteine involves its interaction with specific molecular targets and pathways. It is known to interact with chemotaxis protein CheY in Escherichia coli, influencing bacterial movement. The compound’s phosphonomethyl group plays a crucial role in its binding and activity .
Vergleich Mit ähnlichen Verbindungen
S-Phosphocysteine: Similar in structure but lacks the methyl group.
Phosphinothricin: Contains a phosphinate group instead of a phosphonomethyl group.
2-Amino-3-phosphonopropionic acid: An antagonist of the metabotropic glutamate receptor.
Uniqueness: S-Methyl Phosphocysteine is unique due to its specific phosphonomethyl group, which imparts distinct chemical reactivity and biological activity compared to other similar compounds .
Eigenschaften
CAS-Nummer |
152269-49-3 |
---|---|
Molekularformel |
C4H10NO5PS |
Molekulargewicht |
215.17 g/mol |
IUPAC-Name |
(2R)-2-amino-3-(phosphonomethylsulfanyl)propanoic acid |
InChI |
InChI=1S/C4H10NO5PS/c5-3(4(6)7)1-12-2-11(8,9)10/h3H,1-2,5H2,(H,6,7)(H2,8,9,10)/t3-/m0/s1 |
InChI-Schlüssel |
IIALWEPLPCANHU-VKHMYHEASA-N |
Isomerische SMILES |
C([C@@H](C(=O)O)N)SCP(=O)(O)O |
SMILES |
C(C(C(=O)O)N)SCP(=O)(O)O |
Kanonische SMILES |
C(C(C(=O)O)N)SCP(=O)(O)O |
Synonyme |
2-amino-3-((phosphonomethyl)thio)propionic acid APMTP L-2-amino-3-((phosphonomethyl)thio)propanoic acid |
Herkunft des Produkts |
United States |
Haftungsausschluss und Informationen zu In-Vitro-Forschungsprodukten
Bitte beachten Sie, dass alle Artikel und Produktinformationen, die auf BenchChem präsentiert werden, ausschließlich zu Informationszwecken bestimmt sind. Die auf BenchChem zum Kauf angebotenen Produkte sind speziell für In-vitro-Studien konzipiert, die außerhalb lebender Organismen durchgeführt werden. In-vitro-Studien, abgeleitet von dem lateinischen Begriff "in Glas", beinhalten Experimente, die in kontrollierten Laborumgebungen unter Verwendung von Zellen oder Geweben durchgeführt werden. Es ist wichtig zu beachten, dass diese Produkte nicht als Arzneimittel oder Medikamente eingestuft sind und keine Zulassung der FDA für die Vorbeugung, Behandlung oder Heilung von medizinischen Zuständen, Beschwerden oder Krankheiten erhalten haben. Wir müssen betonen, dass jede Form der körperlichen Einführung dieser Produkte in Menschen oder Tiere gesetzlich strikt untersagt ist. Es ist unerlässlich, sich an diese Richtlinien zu halten, um die Einhaltung rechtlicher und ethischer Standards in Forschung und Experiment zu gewährleisten.