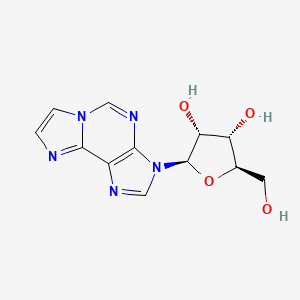
1,N6-Ethenoadenosin
Übersicht
Beschreibung
1,N6-Ethenoadenosine is a modified nucleoside analog that features an additional fused heterocyclic ring of the “etheno” type. This compound is derived from adenosine, a nucleoside that plays a crucial role in various biological processes. The modification involves the addition of an etheno group, which significantly alters its chemical and biological properties .
Wissenschaftliche Forschungsanwendungen
1,N6-Ethenoadenosine has a wide range of scientific research applications, including:
Chemistry: Used as a fluorescent probe to study nucleic acid interactions and enzyme kinetics.
Biology: Employed in the investigation of DNA repair mechanisms and mutagenesis.
Medicine: Explored for its potential as an antiviral and anticancer agent due to its ability to interfere with nucleic acid synthesis.
Industry: Utilized in the development of diagnostic assays and therapeutic agents
Wirkmechanismus
Target of Action
The primary target of 1,N6-Ethenoadenosine is Endonuclease III , an enzyme found in Helicobacter pylori . This enzyme plays a crucial role in DNA repair and replication, making it a significant target for the compound.
Mode of Action
1,N6-Ethenoadenosine is a purine nucleoside analogue . It interacts with its target by inhibiting DNA synthesis and inducing apoptosis . This interaction results in changes to the normal functioning of the cell, particularly in the replication process.
Biochemical Pathways
1,N6-Ethenoadenosine affects the DNA synthesis pathway . By inhibiting DNA synthesis, it disrupts the normal replication process, leading to cell death or apoptosis . This can have downstream effects on cell proliferation and growth, particularly in cancerous cells.
Pharmacokinetics
Like other purine nucleoside analogues, its absorption, distribution, metabolism, and excretion (adme) properties would likely impact its bioavailability and efficacy .
Result of Action
The primary result of 1,N6-Ethenoadenosine’s action is the induction of apoptosis, or programmed cell death . This occurs as a result of the compound’s inhibition of DNA synthesis . This can lead to a decrease in the proliferation of cancerous cells, contributing to its antitumor activity .
Biochemische Analyse
Biochemical Properties
1,N6-Ethenoadenosine plays a significant role in biochemical reactions, particularly in the context of DNA damage and repair mechanisms. It interacts with various enzymes and proteins, including DNA polymerases and glycosylases. For instance, 1,N6-Ethenoadenosine is recognized and excised by the enzyme AlkB in Escherichia coli, which repairs the DNA by removing the adduct . Additionally, it has been shown to interact with protein kinase systems, although no significant biological difference was observed between cyclic adenosine 3’,5’-monophosphate and its fluorescent analog, 1,N6-Ethenoadenosine cyclic 3’,5’-monophosphate .
Cellular Effects
1,N6-Ethenoadenosine has profound effects on cellular processes, particularly in terms of genomic stability. It can promote replication stress and genomic instability by generating extensive frameshifts during translesion synthesis, mediated by human DNA polymerase . This can lead to mutagenic potential and alter the reading frame in mRNA transcripts, contributing to genomic instability . Furthermore, 1,N6-Ethenoadenosine has been implicated in various diseases due to its ability to diminish genome integrity .
Molecular Mechanism
At the molecular level, 1,N6-Ethenoadenosine exerts its effects through binding interactions with biomolecules and enzyme inhibition or activation. It can act as a reverse transcriptase in the presence of damaged ribonucleotides, favoring the addition of deoxypurines opposite the adduct . The compound is also recognized by RNase H2, although it has limited incision activity across the lesion, leading to the persistence of the DNA adduct . This persistence can result in mutagenic outcomes and genomic instability.
Temporal Effects in Laboratory Settings
In laboratory settings, the effects of 1,N6-Ethenoadenosine can change over time. Studies have shown that the compound can generate extensive frameshifts during translesion synthesis, leading to genomic instability . The stability and degradation of 1,N6-Ethenoadenosine in DNA can influence its long-term effects on cellular function. For example, the persistence of the adduct in DNA can lead to prolonged genomic instability and potential mutagenic outcomes .
Dosage Effects in Animal Models
The effects of 1,N6-Ethenoadenosine vary with different dosages in animal models. High doses of the compound have been associated with increased mutagenic potential and genomic instability . In Escherichia coli, 1,N6-Ethenoadenosine was found to be highly mutagenic, causing predominantly A→T transversion mutations . These findings suggest that the compound’s effects are dose-dependent, with higher doses leading to more pronounced adverse effects.
Metabolic Pathways
1,N6-Ethenoadenosine is involved in metabolic pathways related to DNA repair and damage. It is generated endogenously by lipid peroxidation and exogenously by exposure to environmental carcinogens . The compound is recognized and excised by DNA glycosylases, such as AlkB in Escherichia coli, which play a crucial role in repairing the DNA adduct . The metabolism of 1,N6-Ethenoadenosine involves its recognition and removal by specific enzymes, contributing to the maintenance of genomic stability.
Transport and Distribution
Within cells and tissues, 1,N6-Ethenoadenosine is transported and distributed through various mechanisms. It can be recognized by specific transporters and binding proteins that facilitate its localization and accumulation in certain cellular compartments . The compound’s distribution within cells can influence its activity and function, particularly in the context of DNA damage and repair processes.
Subcellular Localization
1,N6-Ethenoadenosine exhibits specific subcellular localization patterns that affect its activity and function. The compound can be directed to specific compartments or organelles through targeting signals or post-translational modifications . Its localization within the cell can influence its interactions with biomolecules and its role in DNA damage and repair mechanisms.
Vorbereitungsmethoden
Synthetic Routes and Reaction Conditions: 1,N6-Ethenoadenosine can be synthesized through the reaction of adenosine with α-halocarbonyl compounds. The process involves the formation of an additional five-membered ring of the imidazole type. This reaction typically requires the presence of bifunctional reagents such as chloroacetaldehyde .
Industrial Production Methods: While specific industrial production methods for 1,N6-Ethenoadenosine are not extensively documented, the general approach involves large-scale synthesis using the same principles as laboratory synthesis. The reaction conditions are optimized for higher yields and purity, often involving advanced purification techniques such as chromatography .
Analyse Chemischer Reaktionen
Types of Reactions: 1,N6-Ethenoadenosine undergoes various chemical reactions, including:
Oxidation: This reaction involves the addition of oxygen or the removal of hydrogen, often using oxidizing agents like hydrogen peroxide.
Reduction: This reaction involves the addition of hydrogen or the removal of oxygen, typically using reducing agents such as sodium borohydride.
Substitution: This reaction involves the replacement of one functional group with another, often using nucleophilic or electrophilic reagents.
Common Reagents and Conditions:
Oxidation: Hydrogen peroxide, under mild acidic conditions.
Reduction: Sodium borohydride, in an aqueous or alcoholic medium.
Substitution: Nucleophilic reagents like ammonia or electrophilic reagents like alkyl halides.
Major Products: The major products formed from these reactions depend on the specific conditions and reagents used. For example, oxidation may yield ethenoadenosine derivatives with additional oxygen-containing functional groups, while reduction may produce simpler adenosine analogs .
Vergleich Mit ähnlichen Verbindungen
- 1,N4-Ethenocytidine
- 1,N2-Ethenoguanosine
- N2,3-Ethenoguanosine
Comparison: 1,N6-Ethenoadenosine is unique due to its specific modification at the N6 position of adenosine, which imparts distinct chemical and biological properties. Compared to other etheno derivatives, it exhibits different fluorescence characteristics and mutagenic potential, making it particularly useful in specific research applications .
Biologische Aktivität
1,N6-Ethenoadenosine (εA) is a significant DNA lesion that arises from the reaction of adenine with various electrophiles, particularly vinyl chloride and lipid peroxidation products. Its biological activity is critical in understanding mutagenesis, DNA repair mechanisms, and its implications in cancer and other diseases. This article synthesizes current research findings, including case studies and analytical data, to provide a comprehensive overview of εA's biological activity.
Formation and Properties of 1,N6-Ethenoadenosine
1,N6-Ethenoadenosine is formed through the alkylation of adenine, leading to a modification that alters its base pairing properties. This modification can result in miscoding during DNA replication, primarily causing A→T transversions along with A→G and A→C mutations. The mutagenic potential of εA has been linked to hotspots for p53 mutations in various cancers, indicating its role in genomic instability .
Table 1: Formation Pathways of 1,N6-Ethenoadenosine
Source | Mechanism | Reference |
---|---|---|
Vinyl Chloride | Direct alkylation of adenine | |
Lipid Peroxidation Products | Reaction with reactive aldehydes | |
Metabolic Activation | Epoxide formation |
Mutagenesis and Cancer
The mutagenic effects of εA are particularly concerning due to their association with cancer development. Studies have shown that cells exposed to εA exhibit increased mutation rates, which can lead to oncogenic transformations. For example, research indicated that εA lesions contribute significantly to mutations in genes associated with tumor suppressor functions, such as p53 .
Repair Mechanisms
Cells possess several mechanisms to repair DNA lesions like εA. The primary pathways include:
- Base Excision Repair (BER) : This pathway recognizes and removes damaged bases, including εA. It involves the action of glycosylases that specifically target modified nucleotides.
- Direct Reversal Repair (DRR) : Some enzymes can directly reverse the modifications caused by εA without excising the base.
Research has demonstrated that while BER is effective against εA, the efficiency can vary among different cell types, influencing overall genomic stability .
Case Studies
Several studies have explored the impact of εA on cellular processes:
- Impact on Translesion Synthesis : A study showed that human DNA polymerase η (hpol η) preferentially incorporates dATP opposite εA during translesion synthesis, which can lead to frameshift mutations. This process highlights the enzyme's dual role as both a DNA polymerase and a reverse transcriptase under conditions where εA is present .
- In Vivo Studies : Research involving animal models exposed to vinyl chloride demonstrated altered protein synthesis patterns due to RNA modifications by εA. These alterations were linked to changes in mRNA translation fidelity, resulting in the production of aberrant proteins .
Table 2: Summary of Case Studies on 1,N6-Ethenoadenosine
Eigenschaften
IUPAC Name |
(2R,3S,4R,5R)-2-(hydroxymethyl)-5-imidazo[2,1-f]purin-3-yloxolane-3,4-diol | |
---|---|---|
Source | PubChem | |
URL | https://pubchem.ncbi.nlm.nih.gov | |
Description | Data deposited in or computed by PubChem | |
InChI |
InChI=1S/C12H13N5O4/c18-3-6-8(19)9(20)12(21-6)17-5-14-7-10-13-1-2-16(10)4-15-11(7)17/h1-2,4-6,8-9,12,18-20H,3H2/t6-,8-,9-,12-/m1/s1 | |
Source | PubChem | |
URL | https://pubchem.ncbi.nlm.nih.gov | |
Description | Data deposited in or computed by PubChem | |
InChI Key |
LRPBXXZUPUBCAP-WOUKDFQISA-N | |
Source | PubChem | |
URL | https://pubchem.ncbi.nlm.nih.gov | |
Description | Data deposited in or computed by PubChem | |
Canonical SMILES |
C1=CN2C=NC3=C(C2=N1)N=CN3C4C(C(C(O4)CO)O)O | |
Source | PubChem | |
URL | https://pubchem.ncbi.nlm.nih.gov | |
Description | Data deposited in or computed by PubChem | |
Isomeric SMILES |
C1=CN2C=NC3=C(C2=N1)N=CN3[C@H]4[C@@H]([C@@H]([C@H](O4)CO)O)O | |
Source | PubChem | |
URL | https://pubchem.ncbi.nlm.nih.gov | |
Description | Data deposited in or computed by PubChem | |
Molecular Formula |
C12H13N5O4 | |
Source | PubChem | |
URL | https://pubchem.ncbi.nlm.nih.gov | |
Description | Data deposited in or computed by PubChem | |
DSSTOX Substance ID |
DTXSID40959846 | |
Record name | 3-Pentofuranosyl-3H-imidazo[2,1-i]purine | |
Source | EPA DSSTox | |
URL | https://comptox.epa.gov/dashboard/DTXSID40959846 | |
Description | DSSTox provides a high quality public chemistry resource for supporting improved predictive toxicology. | |
Molecular Weight |
291.26 g/mol | |
Source | PubChem | |
URL | https://pubchem.ncbi.nlm.nih.gov | |
Description | Data deposited in or computed by PubChem | |
CAS No. |
39007-51-7 | |
Record name | 1,N(6)-Ethenoadenosine | |
Source | ChemIDplus | |
URL | https://pubchem.ncbi.nlm.nih.gov/substance/?source=chemidplus&sourceid=0039007517 | |
Description | ChemIDplus is a free, web search system that provides access to the structure and nomenclature authority files used for the identification of chemical substances cited in National Library of Medicine (NLM) databases, including the TOXNET system. | |
Record name | 3-Pentofuranosyl-3H-imidazo[2,1-i]purine | |
Source | EPA DSSTox | |
URL | https://comptox.epa.gov/dashboard/DTXSID40959846 | |
Description | DSSTox provides a high quality public chemistry resource for supporting improved predictive toxicology. | |
Retrosynthesis Analysis
AI-Powered Synthesis Planning: Our tool employs the Template_relevance Pistachio, Template_relevance Bkms_metabolic, Template_relevance Pistachio_ringbreaker, Template_relevance Reaxys, Template_relevance Reaxys_biocatalysis model, leveraging a vast database of chemical reactions to predict feasible synthetic routes.
One-Step Synthesis Focus: Specifically designed for one-step synthesis, it provides concise and direct routes for your target compounds, streamlining the synthesis process.
Accurate Predictions: Utilizing the extensive PISTACHIO, BKMS_METABOLIC, PISTACHIO_RINGBREAKER, REAXYS, REAXYS_BIOCATALYSIS database, our tool offers high-accuracy predictions, reflecting the latest in chemical research and data.
Strategy Settings
Precursor scoring | Relevance Heuristic |
---|---|
Min. plausibility | 0.01 |
Model | Template_relevance |
Template Set | Pistachio/Bkms_metabolic/Pistachio_ringbreaker/Reaxys/Reaxys_biocatalysis |
Top-N result to add to graph | 6 |
Feasible Synthetic Routes
Haftungsausschluss und Informationen zu In-Vitro-Forschungsprodukten
Bitte beachten Sie, dass alle Artikel und Produktinformationen, die auf BenchChem präsentiert werden, ausschließlich zu Informationszwecken bestimmt sind. Die auf BenchChem zum Kauf angebotenen Produkte sind speziell für In-vitro-Studien konzipiert, die außerhalb lebender Organismen durchgeführt werden. In-vitro-Studien, abgeleitet von dem lateinischen Begriff "in Glas", beinhalten Experimente, die in kontrollierten Laborumgebungen unter Verwendung von Zellen oder Geweben durchgeführt werden. Es ist wichtig zu beachten, dass diese Produkte nicht als Arzneimittel oder Medikamente eingestuft sind und keine Zulassung der FDA für die Vorbeugung, Behandlung oder Heilung von medizinischen Zuständen, Beschwerden oder Krankheiten erhalten haben. Wir müssen betonen, dass jede Form der körperlichen Einführung dieser Produkte in Menschen oder Tiere gesetzlich strikt untersagt ist. Es ist unerlässlich, sich an diese Richtlinien zu halten, um die Einhaltung rechtlicher und ethischer Standards in Forschung und Experiment zu gewährleisten.