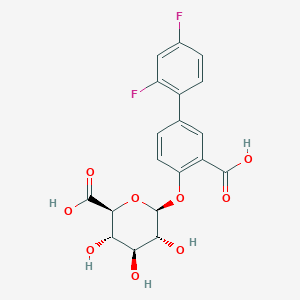
Diflunisal glucuronide ether
Übersicht
Beschreibung
Diflunisal glucuronide ether is a major phase II metabolite of diflunisal, a nonsteroidal anti-inflammatory drug (NSAID). Diflunisal undergoes glucuronidation via uridine diphosphate-glucuronosyltransferase (UGT) enzymes, forming two primary conjugates: acyl glucuronide (DAG) and phenolic glucuronide (DPG). The ether glucuronide refers specifically to the phenolic glucuronide, where the glucuronic acid is attached via an ether bond to the phenolic hydroxyl group of diflunisal .
Vorbereitungsmethoden
Synthetic Routes and Reaction Conditions: The synthesis of diflunisal glucuronide ether involves the glucuronidation of diflunisal. This process typically occurs in the liver, where diflunisal undergoes phase II metabolism to form glucuronide conjugates. The reaction conditions for glucuronidation include the presence of uridine diphosphate glucuronic acid and the enzyme UDP-glucuronosyltransferase .
Industrial Production Methods: Industrial production of this compound is not commonly practiced as it is primarily a metabolic product. the synthesis can be replicated in vitro using liver microsomes or recombinant enzymes to facilitate the glucuronidation process .
Analyse Chemischer Reaktionen
Hydrolysis Reactions
DGE undergoes hydrolysis under specific conditions, regenerating the parent compound and glucuronic acid:
-
Mechanistic Insight : Hydrolysis occurs via cleavage of the β-1-O-ether bond, with rates influenced by steric and electronic factors .
-
Biological Relevance : In vivo hydrolysis contributes to enterohepatic recirculation of diflunisal .
Oxidation and Reduction
DGE participates in redox reactions due to its phenolic and glucuronide moieties:
Reaction Type | Reagents/Conditions | Major Products |
---|---|---|
Oxidation | H₂O₂, oxidative enzymes | Oxidized diflunisal derivatives |
Reduction | NaBH₄, catalytic hydrogenation | Reduced glucuronide intermediates |
-
Oxidation Pathways : The phenolic ring undergoes hydroxylation, forming quinone-like species under strong oxidizing conditions .
-
Reduction Stability : The glucuronide’s carbonyl group shows moderate susceptibility to reducing agents.
Isomerization and Acyl Migration
DGE undergoes pH-dependent intramolecular rearrangements:
Isomer Type | Formation Conditions | Stability |
---|---|---|
α-1-O-acyl isomer | pH 7.4, 37°C | Highly reactive, short-lived |
β-2/3/4-O-acyl isomers | Physiological pH | Stable, accumulate over time |
-
Key Finding : The α-1-O-acyl isomer reversibly converts to β-2/3-O-acyl isomers, challenging earlier assumptions of irreversibility .
-
Analytical Evidence : HPLC/¹H NMR studies confirm isomerization pathways .
Enzymatic Influences on Reactivity
UGT enzymes and inducers modulate DGE’s metabolic fate:
Enzyme Inducer | Effect on DGE Metabolism | Study Model |
---|---|---|
Phenobarbital (PB) | ↑ Ether glucuronidation by 3× | Rat hepatocytes |
β-Naphthoflavone | Mixed induction of UGT isoforms | Human microsomes |
-
Species Variability : Rats show higher acyl glucuronide elimination (~50% dose) compared to humans .
Biological Interactions and Toxicity
Reactive DGE metabolites form covalent adducts with biomolecules:
Interaction Type | Target Molecules | Consequence |
---|---|---|
Transacylation | Serum albumin | Hapten formation, immunogenicity |
Glycation | Hepatic proteins | Cellular dysfunction |
-
Cytotoxicity : DGE’s protein adducts correlate with elevated ALT levels in hepatocyte models .
-
Detoxification : Glutathione conjugation mitigates oxidative damage from DGE-derived quinones .
Comparative Reactivity of Glucuronide Metabolites
DGE’s stability differs from other NSAID glucuronides:
Compound | Half-life (pH 7.4) | Protein Adduct Formation |
---|---|---|
Diflunisal acyl glucuronide | 4.2 hours | High |
DGE (ether glucuronide) | >24 hours | Moderate |
Ibuprofen acyl glucuronide | 1.8 hours | High |
Wissenschaftliche Forschungsanwendungen
Metabolism and Pharmacokinetics
Diflunisal is primarily metabolized into several conjugates, including acyl glucuronides and phenolic glucuronides (ether glucuronides). The metabolic pathways of diflunisal include:
- Acyl Glucuronides : These are unstable and can undergo hydrolysis, leading to the regeneration of diflunisal. They also rearrange into various isomers .
- Phenolic Glucuronides : These metabolites are stable at physiological pH and represent a significant pathway for diflunisal metabolism. Studies indicate that the formation of phenolic glucuronides is capacity-limited, which can affect the drug's pharmacokinetics .
The presence of diflunisal glucuronide ether in biological fluids has been studied extensively using high-performance liquid chromatography (HPLC) and mass spectrometry techniques, providing insights into its pharmacokinetic profile in humans . Understanding these metabolic pathways is crucial for predicting the drug's efficacy and safety.
Toxicological Implications
Diflunisal and its metabolites can lead to gastrointestinal injuries, particularly when administered in high doses. Research indicates that NSAIDs, including diflunisal, can cause mucosal ulceration and bleeding due to their effects on intestinal permeability and inflammation . The role of this compound in these toxicological outcomes is an area of active investigation.
- Mechanism of Injury : The mechanism involves the biliary excretion of glucuronidated NSAIDs into the intestine, where bacterial β-glucuronidases convert them back to their active forms, potentially leading to cellular damage .
Drug Formulation and Bioequivalence Studies
This compound has been utilized in bioequivalence studies to assess the pharmacokinetic similarity between different formulations of diflunisal. A sensitive LC-MS method was developed for the determination of diflunisal and its glucuronide metabolites in human plasma, demonstrating high precision and reliability . This method is essential for:
- Regulatory Approval : Ensuring that new formulations meet safety and efficacy standards.
- Clinical Trials : Monitoring drug levels in subjects during clinical investigations.
Case Studies
Several case studies have highlighted the significance of this compound in clinical settings:
- Case Study 1 : A study on healthy male subjects demonstrated the pharmacokinetic behavior of diflunisal following administration of a 500 mg tablet formulation. The detection limits for diflunisal were established at 0.10 µg/mL, with a linear dynamic range up to 160 µg/mL .
- Case Study 2 : Another investigation explored the differential effects of enzyme inducers on the metabolism of diflunisal. Phenobarbital treatment resulted in increased clearance rates for ether glucuronides, suggesting that enzyme induction can significantly influence drug metabolism .
Summary Table of Applications
Wirkmechanismus
The mechanism of action of diflunisal glucuronide ether is primarily related to its parent compound, diflunisal. Diflunisal inhibits the enzyme cyclooxygenase, thereby reducing the synthesis of prostaglandins, which are mediators of inflammation and pain . The glucuronide conjugation enhances the solubility and excretion of diflunisal, facilitating its elimination from the body .
Vergleich Mit ähnlichen Verbindungen
Key Characteristics:
- Structure : The glucuronide moiety is linked to the 4'-hydroxyl group of diflunisal’s biphenyl ring via an ether bond .
- Metabolic Stability : Unlike the reactive acyl glucuronide (which forms covalent protein adducts), the ether glucuronide is chemically stable and excreted primarily in bile .
- Excretion : In rats, ~12.1% of a diflunisal dose is excreted as ether glucuronide in bile, compared to 27.8% as ester (acyl) glucuronide .
Comparison with Similar Glucuronide Conjugates
Table 1: Structural and Functional Comparison of Glucuronides
Reactivity and Stability
- Acyl vs. Ether Glucuronides: Acyl glucuronides (e.g., diflunisal DAG) undergo hydrolysis, isomerization, and covalent binding to proteins, contributing to toxicity . For example, diflunisal acyl glucuronide has a plasma half-life of 17.3 minutes due to rapid hydrolysis, while its ether glucuronide is more stable .
Morphine Glucuronides :
Metabolic Pathways and Enzyme Induction
- UGT Isoenzyme Specificity: Diflunisal ether glucuronidation is preferentially induced by phenobarbital (3-fold increase in clearance) compared to ester glucuronidation (2-fold increase), suggesting involvement of distinct UGT isoforms . In contrast, 3-methylcholanthrene (3-MC) and β-naphthoflavone (BNF) reduce biliary clearance of both conjugates .
Enterohepatic Recirculation :
Analytical Challenges
Differentiation of Isomers :
Quantification in Biological Matrices :
Toxicological Implications
Acyl Glucuronide Toxicity :
- Ether Glucuronide Safety: Ether-linked conjugates like diflunisal DPG or ethyl glucuronide are non-toxic but may release aglycones under specific conditions (e.g., β-glucuronidase activity in gut microbiota) .
Biologische Aktivität
Diflunisal glucuronide ether, a metabolite of the non-steroidal anti-inflammatory drug (NSAID) diflunisal, has garnered attention due to its biological activity and pharmacokinetics. This article explores its biological properties, metabolic pathways, and potential therapeutic implications based on diverse research findings.
Chemical Structure and Metabolism
This compound is characterized by the molecular formula and is primarily formed through the glucuronidation of diflunisal, which is known for its analgesic and anti-inflammatory effects. The metabolism of diflunisal involves several pathways, leading to various conjugates, including acyl glucuronides and ether glucuronides. These metabolites are crucial for understanding the drug's pharmacological profile and safety.
Analgesic and Anti-Inflammatory Properties
Diflunisal itself exhibits significant analgesic and anti-inflammatory effects. Its glucuronide metabolites, particularly this compound, retain some of these properties. Studies indicate that the glucuronidation process enhances the solubility and bioavailability of diflunisal, allowing for more effective therapeutic action in vivo .
Metabolic Pathways
Research has shown that diflunisal is metabolized primarily to its acyl glucuronide (DAG), phenolic glucuronide (DPG), and sulfate conjugates. The ether glucuronide is excreted in urine, indicating its role in drug elimination . The metabolic pathways involve hydrolysis and rearrangement, which can regenerate diflunisal from its acyl glucuronide form .
Case Study: Human Metabolism
A study involving human subjects demonstrated that after administration of diflunisal, a significant portion was excreted as this compound. The research utilized radiolabeled glucose to trace metabolic pathways, revealing that this metabolite plays a role in hepatic glucose metabolism .
Safety and Toxicity
The safety profile of this compound appears favorable. In clinical settings, diflunisal has been administered in gram quantities without severe adverse effects, suggesting that its metabolites are well tolerated . However, ongoing research is necessary to fully elucidate any long-term effects associated with chronic use.
Implications for Therapeutics
The biological activity of this compound suggests potential therapeutic applications beyond pain management. Its ability to modulate inflammatory responses could be beneficial in treating conditions such as arthritis or other inflammatory diseases. Furthermore, understanding its pharmacokinetics could lead to improved dosing strategies that maximize efficacy while minimizing side effects.
Q & A
Basic Research Questions
Q. What analytical methods are recommended for quantifying diflunisal glucuronide ether and distinguishing it from other conjugates?
To quantify this compound and differentiate it from ester glucuronides or parent compounds, high-performance liquid chromatography (HPLC) coupled with tandem mass spectrometry (MS/MS) is optimal. For example, reversed-phase HPLC with UV detection (e.g., 254 nm) can separate ether and ester glucuronides based on retention times, while MS/MS provides structural confirmation via fragmentation patterns . Specific protocols should include enzymatic hydrolysis controls (e.g., β-glucuronidase treatment) to validate glucuronide identity and exclude interference from non-conjugated metabolites .
Q. How does diflunisal’s metabolism to ether glucuronide impact its pharmacokinetic profile?
Diflunisal undergoes extensive hepatic glucuronidation, with the ether (phenolic) glucuronide accounting for ~90% of urinary metabolites in humans. This pathway is saturable at higher doses, leading to nonlinear pharmacokinetics. Methodologically, radiolabeled diflunisal (e.g., ¹⁴C) can trace the metabolic fate: after administration, urine samples are analyzed for glucuronide conjugates via liquid scintillation counting and chromatographic separation. This approach revealed that 65% of the drug undergoes enterohepatic recirculation, prolonging its half-life .
Advanced Research Questions
Q. How can isotopic labeling resolve controversies in hepatic glucose metabolism using this compound as a tracer?
this compound’s formation from hepatic UDP-glucose allows it to serve as a tracer for glucose-6-phosphate (G6P) pool dynamics. In one study, subjects received ¹⁴C-labeled glucose and diflunisal; urinary glucuronide was hydrolyzed, and its glucose moiety was degraded to quantify ¹⁴C distribution in carbon positions. This revealed that ≤65% of hepatic glycogen derives from the direct pathway (glucose → G6P → glycogen), with the remainder via indirect pathways (e.g., gluconeogenesis). Isotopic labeling paired with hepatic vein catheterization can validate assumptions about G6P pool homogeneity .
Q. What experimental designs mitigate challenges in studying diflunisal acyl glucuronide reactivity and protein adduct formation?
Acyl glucuronides (e.g., diflunisal’s ester conjugate) are chemically reactive and form covalent adducts with serum albumin, complicating stability assessments. To isolate ether glucuronide-specific effects:
- Use in vitro incubation of synthetic ether glucuronide with human serum albumin (HSA) under physiological conditions (pH 7.4, 37°C).
- Monitor adduct formation via LC-MS/MS, targeting signature peptide modifications (e.g., Lys⁴¹³ adduction).
- Compare results to acyl glucuronide incubations, which show time-dependent migration (1-O → 2-, 3-, 4-O isomers) and higher adduct formation .
Q. How do enterohepatic recirculation and renal impairment influence this compound’s pharmacokinetic modeling?
Enterohepatic recirculation (EHC) extends diflunisal’s half-life by reabsorbing cleaved glucuronides. To model this:
- Incorporate a secondary plasma peak (2–4 hours post-dose) and biliary excretion parameters.
- In renal impairment, adjust clearance rates for the ether glucuronide (which accumulates 3-fold in severe cases). Compartmental models should integrate glomerular filtration rate (GFR) and tubular secretion data, validated against plasma and urine LC-MS/MS measurements .
Q. Data Contradictions and Resolution
- Saturable vs. Linear Glucuronidation: notes saturable ether glucuronidation at high doses, while attributes this to EHC. Resolution: Dose-ranging studies (10–500 mg) with frequent plasma sampling can clarify saturation thresholds .
- Hepatic G6P Pool Homogeneity: assumes homogeneity, but tissue-specific isotope dilution may occur. Resolution: Compare ¹⁴C randomization in hepatic vein blood vs. urinary glucuronide under glucagon stimulation .
Eigenschaften
IUPAC Name |
(2S,3S,4S,5R,6S)-6-[2-carboxy-4-(2,4-difluorophenyl)phenoxy]-3,4,5-trihydroxyoxane-2-carboxylic acid | |
---|---|---|
Source | PubChem | |
URL | https://pubchem.ncbi.nlm.nih.gov | |
Description | Data deposited in or computed by PubChem | |
InChI |
InChI=1S/C19H16F2O9/c20-8-2-3-9(11(21)6-8)7-1-4-12(10(5-7)17(25)26)29-19-15(24)13(22)14(23)16(30-19)18(27)28/h1-6,13-16,19,22-24H,(H,25,26)(H,27,28)/t13-,14-,15+,16-,19+/m0/s1 | |
Source | PubChem | |
URL | https://pubchem.ncbi.nlm.nih.gov | |
Description | Data deposited in or computed by PubChem | |
InChI Key |
RBZXVQOCMCPTHC-KSPMYQCISA-N | |
Source | PubChem | |
URL | https://pubchem.ncbi.nlm.nih.gov | |
Description | Data deposited in or computed by PubChem | |
Canonical SMILES |
C1=CC(=C(C=C1C2=C(C=C(C=C2)F)F)C(=O)O)OC3C(C(C(C(O3)C(=O)O)O)O)O | |
Source | PubChem | |
URL | https://pubchem.ncbi.nlm.nih.gov | |
Description | Data deposited in or computed by PubChem | |
Isomeric SMILES |
C1=CC(=C(C=C1C2=C(C=C(C=C2)F)F)C(=O)O)O[C@H]3[C@@H]([C@H]([C@@H]([C@H](O3)C(=O)O)O)O)O | |
Source | PubChem | |
URL | https://pubchem.ncbi.nlm.nih.gov | |
Description | Data deposited in or computed by PubChem | |
Molecular Formula |
C19H16F2O9 | |
Source | PubChem | |
URL | https://pubchem.ncbi.nlm.nih.gov | |
Description | Data deposited in or computed by PubChem | |
DSSTOX Substance ID |
DTXSID50207143 | |
Record name | Diflunisal glucuronide ether | |
Source | EPA DSSTox | |
URL | https://comptox.epa.gov/dashboard/DTXSID50207143 | |
Description | DSSTox provides a high quality public chemistry resource for supporting improved predictive toxicology. | |
Molecular Weight |
426.3 g/mol | |
Source | PubChem | |
URL | https://pubchem.ncbi.nlm.nih.gov | |
Description | Data deposited in or computed by PubChem | |
CAS No. |
58446-29-0 | |
Record name | Diflunisal glucuronide ether | |
Source | ChemIDplus | |
URL | https://pubchem.ncbi.nlm.nih.gov/substance/?source=chemidplus&sourceid=0058446290 | |
Description | ChemIDplus is a free, web search system that provides access to the structure and nomenclature authority files used for the identification of chemical substances cited in National Library of Medicine (NLM) databases, including the TOXNET system. | |
Record name | Diflunisal glucuronide ether | |
Source | EPA DSSTox | |
URL | https://comptox.epa.gov/dashboard/DTXSID50207143 | |
Description | DSSTox provides a high quality public chemistry resource for supporting improved predictive toxicology. | |
Haftungsausschluss und Informationen zu In-Vitro-Forschungsprodukten
Bitte beachten Sie, dass alle Artikel und Produktinformationen, die auf BenchChem präsentiert werden, ausschließlich zu Informationszwecken bestimmt sind. Die auf BenchChem zum Kauf angebotenen Produkte sind speziell für In-vitro-Studien konzipiert, die außerhalb lebender Organismen durchgeführt werden. In-vitro-Studien, abgeleitet von dem lateinischen Begriff "in Glas", beinhalten Experimente, die in kontrollierten Laborumgebungen unter Verwendung von Zellen oder Geweben durchgeführt werden. Es ist wichtig zu beachten, dass diese Produkte nicht als Arzneimittel oder Medikamente eingestuft sind und keine Zulassung der FDA für die Vorbeugung, Behandlung oder Heilung von medizinischen Zuständen, Beschwerden oder Krankheiten erhalten haben. Wir müssen betonen, dass jede Form der körperlichen Einführung dieser Produkte in Menschen oder Tiere gesetzlich strikt untersagt ist. Es ist unerlässlich, sich an diese Richtlinien zu halten, um die Einhaltung rechtlicher und ethischer Standards in Forschung und Experiment zu gewährleisten.