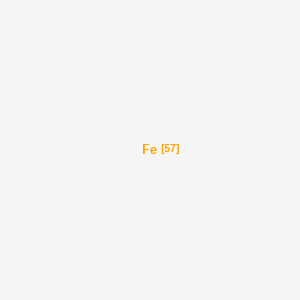
Iron-57
Übersicht
Beschreibung
Iron-57 (⁵⁷Fe) is a stable isotope of iron with a natural abundance of 2.2% . It is distinguished by its nuclear properties, including a low-energy gamma-ray emission (14.4 keV) and a long-lived excited state (98.3 ns), which make it exceptionally suited for Mössbauer spectroscopy . The Mössbauer effect in ⁵⁷Fe enables ultra-high-resolution measurements (1 in 10¹²) due to its narrow natural linewidth (5 × 10⁻⁹ eV), allowing precise characterization of electronic environments, oxidation states, and magnetic interactions in solid-state materials, proteins, and coordination compounds .
Vorbereitungsmethoden
Neutron Activation of Iron-56
The primary industrial method for ⁵⁷Fe production involves neutron capture by Iron-56 (⁵⁶Fe) in nuclear reactors. When ⁵⁶Fe is irradiated with thermal neutrons (energy < 0.025 eV), the following reaction occurs:
This exothermic process leverages the high neutron capture cross-section of ⁵⁶Fe (2.6 barns at 14.8 MeV) . Reactor-grade ⁵⁶Fe targets are enriched to >99% isotopic purity via electromagnetic separation or gas centrifuges prior to irradiation. The yield depends on neutron flux density, irradiation duration, and target geometry. For example, a 100 g ⁵⁶Fe target exposed to a neutron flux of 10¹⁴ n·cm⁻²·s⁻¹ for 30 days achieves ~15% conversion to ⁵⁷Fe .
Isotopic Enrichment via Crystallization
Post-irradiation, the isotopic mixture undergoes fractional crystallization to separate ⁵⁷Fe from unreacted ⁵⁶Fe and other isotopes. This process exploits differences in isotopic partitioning between solid and liquid phases during metal solidification. Experimental studies on iron meteorites provide key insights:
Solid-Liquid Partitioning Dynamics
During crystallization, ⁵⁷Fe preferentially enriches in the liquid phase due to its slightly lower vibrational entropy compared to ⁵⁶Fe. The fractionation factor (α₅₇/₅₆) follows the relationship:
{57/56} = \frac{(^{57}\text{Fe}/^{56}\text{Fe}){\text{solid}}}{(^{57}\text{Fe}/^{56}\text{Fe})_{\text{liquid}}} \approx 0.9995 \, \text{at} \, 1325^\circ\text{C}
Controlled cooling rates (1–5°C·h⁻¹) and temperature gradients optimize separation efficiency. Industrial setups use induction furnaces with inert atmospheres (Ar or He) to prevent oxidation.
Troilite-Assisted Purification
Incorporating sulfur (as FeS or troilite) enhances isotopic separation. At 900–1200°C, ⁵⁷Fe partitions into sulfide phases due to its stronger affinity for S-rich melts. The equilibrium fractionation between metal and troilite is:
This method achieves >98% ⁵⁷Fe purity after three iterative crystallization cycles.
Chromatographic Separation Techniques
High-performance ion-exchange chromatography (HPIEC) is employed for final purification. Key parameters include:
Column Chemistry
-
Resin : Chelating resins functionalized with aminophosphonic acid groups (e.g., Purolite S940)
-
Eluent : 0.5 M HNO₃ with 0.1 M citric acid (pH 1.8)
-
Flow Rate : 2 mL·min⁻¹
Isotopic Resolution
The separation factor (β) between ⁵⁶Fe and ⁵⁷Fe in HPIEC is:
{\text{eluate}}}{(^{57}\text{Fe}/^{56}\text{Fe}){\text{feed}}} = 1.0024 \pm 0.0003
Multi-column cascades with recycle streams achieve 99.9% isotopic purity, suitable for Mössbauer spectroscopy applications .
Quality Control and Analytical Validation
Mass Spectrometric Analysis
Isotopic ratios are verified using multi-collector inductively coupled plasma mass spectrometry (MC-ICP-MS). Typical operating conditions:
Parameter | Value |
---|---|
RF Power | 1350 W |
Plasma Gas Flow | 16 L·min⁻¹ Ar |
Resolution | 4000 (medium) |
Integration Time | 8 s per isotope |
External reproducibility is ±0.05‰ (2σ) for δ⁵⁷Fe .
Mössbauer Spectroscopy
Phase purity and electronic environment are assessed via ⁵⁷Fe Mössbauer spectroscopy. Hyperfine parameters for metallic ⁵⁷Fe:
Parameter | Value |
---|---|
Isomer Shift (δ) | 0.00 ± 0.02 mm·s⁻¹ |
Quadrupole Splitting | 0.00 ± 0.02 mm·s⁻¹ |
Magnetic Hyperfine Field | 33.0 ± 0.1 T |
Deviations >0.05 mm·s⁻¹ indicate contamination or lattice defects .
Industrial-Scale Production Workflows
A representative production flowchart for ⁵⁷Fe is as follows:
-
Target Preparation : ⁵⁶Fe powder (99.99% purity) pressed into 10 cm diameter pellets.
-
Neutron Irradiation : 30-day exposure in a thermal neutron flux of 10¹⁴ n·cm⁻²·s⁻¹.
-
Decay Cooling : 14-day cooldown to allow short-lived radionuclides (e.g., ⁵⁹Fe) to decay.
-
Crystallization : Three-stage fractional crystallization at 1200°C under Ar.
-
Chromatography : Two-pass HPIEC purification.
-
Final Product : ⁵⁷Fe foil (99.9% isotopic purity, 0.1 mm thickness).
Challenges and Mitigation Strategies
Neutron Economy Optimization
To maximize ⁵⁶Fe→⁵⁷Fe conversion:
-
Use moderated neutron spectra (Cd ratio > 100) to reduce epithermal neutron flux.
-
Implement rotating target assemblies for uniform irradiation.
Sulfur Contamination Control
Residual sulfur from troilite-assisted crystallization is removed via:
-
Vacuum distillation at 10⁻⁶ mbar and 800°C.
-
Gettering with Zr foil at 400°C.
Analyse Chemischer Reaktionen
Types of Reactions: Iron-57, like other iron isotopes, can undergo various chemical reactions, including oxidation, reduction, and substitution reactions.
Common Reagents and Conditions:
Oxidation: this compound can be oxidized using oxidizing agents such as oxygen or hydrogen peroxide under controlled conditions.
Reduction: Reduction of this compound can be achieved using reducing agents like hydrogen gas or carbon monoxide.
Major Products Formed: The major products formed from these reactions depend on the specific reagents and conditions used. For example, oxidation of this compound can produce iron oxides, while reduction can yield elemental iron .
Wissenschaftliche Forschungsanwendungen
Chemistry
Iron-57 is extensively utilized in Mössbauer spectroscopy, a technique that allows researchers to investigate the electronic and structural properties of iron-containing compounds. The Mössbauer effect enables the resonant absorption and emission of gamma rays without significant energy loss, providing insights into:
- Electronic Structure : Understanding oxidation states and coordination environments.
- Magnetic Properties : Studying magnetization dynamics on rapid timescales.
Table 1: Key Properties of this compound for Mössbauer Spectroscopy
Property | Value |
---|---|
Gamma-ray Energy | 14.4 keV |
Natural Linewidth | eV |
Resolution Capability |
Biology
In biological research, this compound serves as a tracer to study iron metabolism and transport mechanisms in living organisms. Notable applications include:
- Metabolic Tracer Studies : Used to track iron absorption and excretion, providing insights into genetic control mechanisms related to iron metabolism.
- Anemia Interventions : Research has shown that this compound can help develop effective interventions for iron deficiency anemia.
Case Study: Long-term Iron Absorption in Children
A study conducted on Gambian toddlers demonstrated the use of this compound to measure long-term iron absorption during supplementation. The findings revealed that daily iron absorption increased significantly during the supplementation period, highlighting the isotope's effectiveness in clinical research .
Medicine
This compound is integral to medical research focused on improving iron supplementation strategies. Its applications include:
- Clinical Trials : Assessing the efficiency of iron supplements and their impact on overall health.
- Understanding Iron Balance : Investigating the relationship between iron intake and loss over time.
Table 2: Applications of this compound in Medical Research
Application | Description |
---|---|
Clinical Trials | Evaluating new iron supplementation methods |
Iron Balance Studies | Measuring absorption vs. loss rates |
Industry
In industrial applications, this compound is used for developing new materials and studying catalytic processes. Its properties make it valuable for:
- Material Science : Investigating the structural integrity and performance of metal composites.
- Catalysis Research : Understanding catalytic mechanisms at the atomic level.
Wirkmechanismus
The mechanism by which Iron-57 exerts its effects is primarily through its nuclear properties. In Mössbauer spectroscopy, the isotope’s nuclear transitions are used to probe the local environment of iron atoms in a sample. This technique provides detailed information about the electronic, magnetic, and structural properties of the material being studied .
Vergleich Mit ähnlichen Verbindungen
Comparison with Similar Compounds and Isotopes
Mössbauer-Active Isotopes: Iron-57 vs. Tantalum-181
While ⁵⁷Fe is the most widely used Mössbauer isotope, tantalum-181 (¹⁸¹Ta) is another notable candidate. Key differences include:
¹⁸¹Ta’s narrower linewidth provides finer energy resolution, but its lower gamma-ray energy limits penetration depth in materials compared to ⁵⁷Fe .
Iron Isotopes: ⁵⁴Fe, ⁵⁶Fe, ⁵⁷Fe, and ⁵⁸Fe
Iron has four stable isotopes, each with distinct nuclear and industrial applications:
⁵⁷Fe’s unique nuclear properties contrast with ⁵⁶Fe’s dominance in natural abundance and ⁵⁸Fe’s niche role in radiochemistry.
Gravitational Redshift Measurement (Pound-Rebka Experiment)
The ⁵⁷Fe gamma-ray transition was used to validate Einstein’s general relativity by measuring gravitational redshift in a 22.6-meter vertical tower. The experiment achieved a precision of 1% deviation from theoretical predictions .
Industrial and Medical Relevance
- Cobalt-58m production: Deuteron bombardment of enriched ⁵⁷Fe targets yields ⁵⁸Co, a theranostic isotope, but requires high-purity ⁵⁷Fe to avoid impurities like ⁵⁶Co and ⁵⁷Co .
- Zero-point field studies : ⁵⁷Fe nuclei in X-ray waveguides exhibit accelerated γ-emission under X-ray stimulation, revealing interactions between vacuum fluctuations and nuclear transitions .
Biologische Aktivität
Iron-57 () is a stable isotope of iron that plays significant roles in biological systems, particularly in human physiology. This article explores the biological activity of this compound, focusing on its functions, mechanisms, and implications in health and disease.
Role in Biological Systems
Essential Component of Hemoglobin and Myoglobin
This compound is crucial for the formation of hemoglobin and myoglobin, proteins responsible for oxygen transport and storage in blood and muscle tissues, respectively. These proteins rely on iron for their ability to bind oxygen, thus facilitating cellular respiration and energy production .
Enzymatic Processes
This compound acts as a cofactor in various enzymatic reactions. It is particularly important in the activity of ribonucleotide reductase, an enzyme essential for DNA synthesis. This involvement highlights the isotope's role in cellular proliferation and genetic stability.
Redox Reactions
The ability of this compound to undergo redox reactions is vital for electron transfer processes within cells. This property is critical for metabolic pathways, including those involved in cellular respiration. However, under certain conditions, this compound can contribute to oxidative stress through the Fenton reaction, generating reactive oxygen species (ROS) that may lead to cellular damage.
Case Studies
-
Iron Absorption in Children
A study investigated the long-term absorption and loss of iron using this compound as a tracer in Gambian toddlers. The results indicated that daily iron absorption increased significantly during supplementation with this compound compared to control periods. Specifically, absorption rates rose from 0.26 mg/day to 1.00 mg/day during supplementation, while daily iron loss also increased significantly . -
Heme Isotopic Fine Structure Analysis
Another study utilized Fourier Transform Ion Cyclotron Resonance Mass Spectrometry (FTICR-MS) to analyze the distribution of this compound-labeled heme in mouse spleens. The findings demonstrated the potential of using stable isotopes for tracking dietary iron utilization and localization within tissues . The study highlighted splenomegaly as a response to treatment with C.E.R.A., showing how this compound incorporation can indicate physiological changes associated with erythropoiesis .
Isotope Fractionation
Research indicates that biological processes can fractionate iron isotopes, including . This fractionation provides insights into iron homeostasis and metabolic pathways affecting iron absorption and storage within the body. For instance, variations in isotopic composition have been linked to conditions such as anemia and hemochromatosis .
Interaction with Organic Ligands
Iron's binding to organic ligands influences its isotopic fractionation beyond simple redox reactions. Studies have shown that during anoxic periods, the interaction between dissolved organic carbon (DOC) and iron increases, affecting its bioavailability and utilization by organisms .
Data Summary
The following table summarizes key findings from various studies on this compound:
Q & A
Basic Research Questions
Q. How is Iron-57 utilized in Mössbauer spectroscopy, and what methodological considerations are critical for experimental design?
this compound’s nuclear properties, including a sharp 14.4 keV gamma-ray transition and natural linewidth of 5×10⁻⁹ eV, make it ideal for Mössbauer spectroscopy . Key methodological steps include:
- Sample Preparation : Use thin absorbers (≤10 mg/cm² Fe) to minimize thickness effects, ensuring optimal resonance .
- Calibration : Calibrate velocity drives using α-Fe foil standards to ensure sub-millimeter/second precision .
- Temperature Control : Maintain cryogenic conditions (e.g., 4.2 K) for studying magnetic ordering or lattice dynamics .
- Data Analysis : Fit spectra using Lorentzian line shapes and quantify hyperfine parameters (isomer shift, quadrupole splitting, magnetic hyperfine field) with software like MossWinn or RECOIL .
Q. What experimental protocols ensure reproducibility in studies involving this compound’s Mössbauer effect?
Reproducibility requires:
- Standardized Instrumentation : Use transmission geometry spectrometers with proportional counters or avalanche photodiodes .
- Radiation Safety : Shield ⁵⁷Co sources (common parent isotope) with lead containers to minimize gamma-ray exposure .
- Validation : Cross-check results with complementary techniques like X-ray absorption spectroscopy (XAS) or nuclear magnetic resonance (NMR) .
- Reporting Standards : Document velocity calibration, temperature, and absorber thickness in line with guidelines from journals like Reviews in Analytical Chemistry .
Q. How can researchers design experiments to probe this compound’s role in biological systems, such as hemoglobin?
- Sample Isolation : Purify hemoglobin derivatives (e.g., oxyhemoglobin vs. deoxyhemoglobin) under inert atmospheres to preserve oxidation states .
- Low-Temperature Measurements : Capture ligand-binding dynamics by freezing samples at 77 K .
- Comparative Analysis : Contrast hyperfine parameters of synthetic model compounds (e.g., heme complexes) with native proteins to infer electronic structure changes .
Advanced Research Questions
Q. How can conflicting Mössbauer spectral data for this compound in heterogeneous materials (e.g., mixed-valence oxides) be resolved?
- Multi-Site Fitting : Deconvolute overlapping spectra using software that supports distributed hyperfine parameter models .
- In Situ Characterization : Pair Mössbauer spectroscopy with X-ray diffraction (XRD) under controlled environments (e.g., varying O₂ partial pressures) to correlate phase transitions with spectral changes .
- Synchrotron Validation : Validate ambiguous assignments with synchrotron-based techniques like nuclear forward scattering (NFS) .
Q. What methodologies enable precise measurement of gravitational redshift using this compound, as demonstrated in the Pound-Rebka experiment?
- Resonant Absorption Setup : Place a ⁵⁷Co source at the base of a vertical tower (e.g., 22.6 m) and an ⁵⁷Fe absorber at the top. Use piezoelectric transducers to modulate the source’s velocity and compensate for energy shifts .
- Doppler Calibration : Apply a velocity gradient matching Earth’s gravitational potential (ΔE/E ≈ 2.5×10⁻¹⁵ per meter) .
- Error Mitigation : Correct for thermal broadening by stabilizing the system to ±0.1 K and averaging data over long acquisition times .
Q. How can this compound’s nuclear cross-section data inform neutron scattering experiments in condensed matter physics?
- Cross-Section Libraries : Reference evaluated nuclear data (e.g., ENDF/B-VIII.0) for ⁵⁷Fe’s neutron capture (σ = 2.6 barns at 14.8 MeV) and inelastic scattering profiles .
- Monte Carlo Simulations : Model neutron transport in iron-rich materials (e.g., steels) using MCNP or GEANT4, incorporating ⁵⁷Fe’s isotopic abundance (2.1%) and resonance behavior .
- Benchmarking : Validate simulations against experimental benchmarks, such as time-of-flight spectra from spallation sources .
Q. What advanced statistical frameworks are suitable for analyzing uncertainties in this compound Mössbauer data from disordered systems?
- Bayesian Inference : Use Markov Chain Monte Carlo (MCMC) methods to quantify parameter uncertainties and model selection (e.g., comparing single vs. distributed sites) .
- Principal Component Analysis (PCA) : Reduce dimensionality in datasets with overlapping spectral features (e.g., amorphous alloys) .
- Error Propagation : Apply the Kramers-Kronig relations to account for instrumental broadening effects in linewidth calculations .
Q. Data Presentation and Reproducibility Guidelines
- Tables : Include hyperfine parameters (isomer shift, quadrupole splitting) with errors (e.g., δ = 0.32 ± 0.02 mm/s) and experimental conditions (T, absorber thickness) .
- Figures : Label Mössbauer spectra with velocity scales (±10 mm/s) and annotate subspectra corresponding to distinct iron sites .
- Supplemental Information : Provide raw spectra, calibration curves, and computational scripts in repositories like Zenodo or institutional databases .
Eigenschaften
IUPAC Name |
iron-57 | |
---|---|---|
Source | PubChem | |
URL | https://pubchem.ncbi.nlm.nih.gov | |
Description | Data deposited in or computed by PubChem | |
InChI |
InChI=1S/Fe/i1+1 | |
Source | PubChem | |
URL | https://pubchem.ncbi.nlm.nih.gov | |
Description | Data deposited in or computed by PubChem | |
InChI Key |
XEEYBQQBJWHFJM-OUBTZVSYSA-N | |
Source | PubChem | |
URL | https://pubchem.ncbi.nlm.nih.gov | |
Description | Data deposited in or computed by PubChem | |
Canonical SMILES |
[Fe] | |
Source | PubChem | |
URL | https://pubchem.ncbi.nlm.nih.gov | |
Description | Data deposited in or computed by PubChem | |
Isomeric SMILES |
[57Fe] | |
Source | PubChem | |
URL | https://pubchem.ncbi.nlm.nih.gov | |
Description | Data deposited in or computed by PubChem | |
Molecular Formula |
Fe | |
Source | PubChem | |
URL | https://pubchem.ncbi.nlm.nih.gov | |
Description | Data deposited in or computed by PubChem | |
DSSTOX Substance ID |
DTXSID60933168 | |
Record name | (~57~Fe)Iron | |
Source | EPA DSSTox | |
URL | https://comptox.epa.gov/dashboard/DTXSID60933168 | |
Description | DSSTox provides a high quality public chemistry resource for supporting improved predictive toxicology. | |
Molecular Weight |
56.935392 g/mol | |
Source | PubChem | |
URL | https://pubchem.ncbi.nlm.nih.gov | |
Description | Data deposited in or computed by PubChem | |
CAS No. |
14762-69-7 | |
Record name | Iron, isotope of mass 57 | |
Source | ChemIDplus | |
URL | https://pubchem.ncbi.nlm.nih.gov/substance/?source=chemidplus&sourceid=0014762697 | |
Description | ChemIDplus is a free, web search system that provides access to the structure and nomenclature authority files used for the identification of chemical substances cited in National Library of Medicine (NLM) databases, including the TOXNET system. | |
Record name | (~57~Fe)Iron | |
Source | EPA DSSTox | |
URL | https://comptox.epa.gov/dashboard/DTXSID60933168 | |
Description | DSSTox provides a high quality public chemistry resource for supporting improved predictive toxicology. | |
Synthesis routes and methods I
Procedure details
Synthesis routes and methods II
Procedure details
Synthesis routes and methods III
Procedure details
Haftungsausschluss und Informationen zu In-Vitro-Forschungsprodukten
Bitte beachten Sie, dass alle Artikel und Produktinformationen, die auf BenchChem präsentiert werden, ausschließlich zu Informationszwecken bestimmt sind. Die auf BenchChem zum Kauf angebotenen Produkte sind speziell für In-vitro-Studien konzipiert, die außerhalb lebender Organismen durchgeführt werden. In-vitro-Studien, abgeleitet von dem lateinischen Begriff "in Glas", beinhalten Experimente, die in kontrollierten Laborumgebungen unter Verwendung von Zellen oder Geweben durchgeführt werden. Es ist wichtig zu beachten, dass diese Produkte nicht als Arzneimittel oder Medikamente eingestuft sind und keine Zulassung der FDA für die Vorbeugung, Behandlung oder Heilung von medizinischen Zuständen, Beschwerden oder Krankheiten erhalten haben. Wir müssen betonen, dass jede Form der körperlichen Einführung dieser Produkte in Menschen oder Tiere gesetzlich strikt untersagt ist. Es ist unerlässlich, sich an diese Richtlinien zu halten, um die Einhaltung rechtlicher und ethischer Standards in Forschung und Experiment zu gewährleisten.