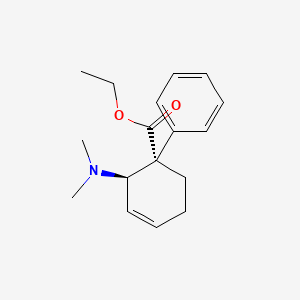
Dextilidin
Übersicht
Beschreibung
Dextilidine is an organic compound classified as an aralkylamine. It is a derivative of ethyl 2-(dimethylamino)-1-phenylcyclohex-3-ene-1-carboxylate. This compound is known for its role as an opioid analgesic, which means it is used to relieve pain by acting on the opioid receptors in the brain .
Wissenschaftliche Forschungsanwendungen
Dextilidine has several scientific research applications, including:
Chemistry: Used as a model compound in studying the reactivity and synthesis of aralkylamines.
Biology: Investigated for its effects on opioid receptors and its potential as a pain-relieving agent.
Medicine: Explored for its analgesic properties and potential therapeutic applications in pain management.
Industry: Utilized in the development of new analgesic drugs and in the study of opioid receptor interactions
Wirkmechanismus
Target of Action
Dextilidine, also known as Tilidine, is an opioid analgesic . The primary targets of Dextilidine are the opiate receptors in the central and peripheral nervous systems . These receptors play a crucial role in suppressing pain perception and transmission .
Mode of Action
Dextilidine interacts with its targets, the opiate receptors, resulting in the suppression of pain perception and transmission . It is the (1S,2R)-isomer (dextilidine) that is responsible for its analgesic activity . .
Biochemical Pathways
As a prodrug, Tilidine is converted by the liver to the active analgesic, nortilidine . Virtually all of the opioid activity resides in the (1S,2R) isomer, i.e., the isomer derived from Dextilidine . The downstream effects of these pathways primarily involve the suppression of pain signals.
Pharmacokinetics
As a prodrug, Tilidine is metabolized in the liver to produce the active analgesic, nortilidine
Result of Action
The molecular and cellular effects of Dextilidine’s action primarily involve the suppression of pain perception and transmission . By interacting with opiate receptors in the central and peripheral nervous systems, Dextilidine helps to alleviate pain .
Action Environment
It is known that various factors, such as the patient’s overall health, liver function (since tilidine is metabolized in the liver to produce the active analgesic, nortilidine ), and the presence of other drugs, can potentially influence the action and efficacy of Dextilidine.
Biochemische Analyse
Biochemical Properties
Dextilidine plays a significant role in biochemical reactions as an opioid analgesic. It interacts with various enzymes, proteins, and other biomolecules. The primary interaction is with the liver enzymes CYP3A4 and CYP2C19, which metabolize dextilidine into its active form, nortilidine . This interaction is crucial for the conversion of dextilidine into its pharmacologically active form. Additionally, dextilidine interacts with opioid receptors in the central nervous system, leading to its analgesic effects .
Cellular Effects
Dextilidine influences various types of cells and cellular processes. It primarily affects neuronal cells by binding to opioid receptors, which are G-protein coupled receptors. This binding inhibits adenylate cyclase activity, reducing the production of cyclic AMP (cAMP) and leading to decreased neuronal excitability . Dextilidine also impacts cell signaling pathways, gene expression, and cellular metabolism by modulating the release of neurotransmitters such as dopamine, serotonin, and norepinephrine .
Molecular Mechanism
The molecular mechanism of dextilidine involves its conversion to nortilidine, which then binds to opioid receptors in the brain and spinal cord . This binding inhibits the release of neurotransmitters and reduces the perception of pain. Dextilidine also acts as a competitive inhibitor of the enzyme adenylate cyclase, leading to decreased levels of cAMP . This reduction in cAMP levels results in the inhibition of protein kinase A (PKA) and subsequent changes in gene expression .
Temporal Effects in Laboratory Settings
In laboratory settings, the effects of dextilidine change over time. Dextilidine is rapidly metabolized in the liver, with its active metabolite, nortilidine, having a half-life of approximately 3-5 hours . The stability of dextilidine is influenced by factors such as temperature and pH, with degradation occurring more rapidly under acidic conditions . Long-term effects on cellular function include potential tolerance and dependence due to chronic exposure to the drug .
Dosage Effects in Animal Models
The effects of dextilidine vary with different dosages in animal models. At low doses, dextilidine produces analgesic effects without significant adverse effects . At higher doses, dextilidine can cause respiratory depression, sedation, and potential toxicity . Threshold effects observed in studies include the development of tolerance and physical dependence with prolonged use .
Metabolic Pathways
Dextilidine is involved in several metabolic pathways. It is primarily metabolized by the liver enzymes CYP3A4 and CYP2C19 to form nortilidine . This active metabolite is further metabolized to bisnortilidine . The metabolic flux of dextilidine involves the conversion of the parent compound to its active and inactive metabolites, which are then excreted in the urine .
Transport and Distribution
Dextilidine is transported and distributed within cells and tissues through passive diffusion and active transport mechanisms . It binds to plasma proteins, which facilitates its distribution throughout the body . Dextilidine’s localization and accumulation are influenced by its lipophilicity, allowing it to cross the blood-brain barrier and exert its effects on the central nervous system .
Subcellular Localization
The subcellular localization of dextilidine is primarily within the cytoplasm and the endoplasmic reticulum of liver cells, where it undergoes metabolic conversion . Dextilidine’s activity and function are influenced by its localization, with the active metabolite nortilidine exerting its effects on opioid receptors in the brain and spinal cord . Post-translational modifications, such as phosphorylation, may also play a role in directing dextilidine to specific cellular compartments .
Vorbereitungsmethoden
Synthetic Routes and Reaction Conditions: Dextilidine is synthesized through a series of chemical reactions involving the starting materials ethyl 2-(dimethylamino)-1-phenylcyclohex-3-ene-1-carboxylateThe reaction conditions typically include the use of organic solvents, catalysts, and controlled temperatures to ensure the desired stereochemistry of the product .
Industrial Production Methods: Industrial production of dextilidine involves large-scale synthesis using optimized reaction conditions to maximize yield and purity. The process includes purification steps such as recrystallization and chromatography to isolate the final product. The production is carried out in compliance with regulatory standards to ensure the safety and efficacy of the compound .
Analyse Chemischer Reaktionen
Types of Reactions: Dextilidine undergoes various chemical reactions, including:
Oxidation: The compound can be oxidized to form corresponding oxides.
Reduction: Reduction reactions can convert dextilidine to its reduced forms.
Substitution: The compound can undergo substitution reactions where functional groups are replaced by other groups.
Common Reagents and Conditions:
Oxidation: Common oxidizing agents include potassium permanganate and chromium trioxide.
Reduction: Reducing agents such as lithium aluminum hydride and sodium borohydride are used.
Substitution: Reagents like halogens and alkylating agents are employed under controlled conditions.
Major Products Formed: The major products formed from these reactions include various derivatives of dextilidine, such as its oxides, reduced forms, and substituted analogs .
Vergleich Mit ähnlichen Verbindungen
Tilidine: A racemate comprising equimolar amounts of dextilidine and its enantiomer, ent-dextilidine.
Nortilidine: The active metabolite of dextilidine, responsible for its analgesic effects.
Comparison: Dextilidine is unique in its stereochemistry, with specific configurations at the carbon atoms bearing the phenyl and dimethylamino groups. This stereochemistry is crucial for its conversion to the active form, nortilidine, which exhibits the desired analgesic properties. Compared to tilidine, dextilidine is more specific in its action due to its defined stereochemistry .
Eigenschaften
CAS-Nummer |
51931-66-9 |
---|---|
Molekularformel |
C17H23NO2 |
Molekulargewicht |
273.37 g/mol |
IUPAC-Name |
ethyl (1R,2S)-2-(dimethylamino)-1-phenylcyclohex-3-ene-1-carboxylate |
InChI |
InChI=1S/C17H23NO2/c1-4-20-16(19)17(14-10-6-5-7-11-14)13-9-8-12-15(17)18(2)3/h5-8,10-12,15H,4,9,13H2,1-3H3/t15-,17+/m0/s1 |
InChI-Schlüssel |
WDEFBBTXULIOBB-DOTOQJQBSA-N |
SMILES |
CCOC(=O)C1(CCC=CC1N(C)C)C2=CC=CC=C2 |
Isomerische SMILES |
CCOC(=O)[C@]1(CCC=C[C@@H]1N(C)C)C2=CC=CC=C2 |
Kanonische SMILES |
CCOC(=O)C1(CCC=CC1N(C)C)C2=CC=CC=C2 |
20380-58-9 | |
Piktogramme |
Irritant |
Herkunft des Produkts |
United States |
Retrosynthesis Analysis
AI-Powered Synthesis Planning: Our tool employs the Template_relevance Pistachio, Template_relevance Bkms_metabolic, Template_relevance Pistachio_ringbreaker, Template_relevance Reaxys, Template_relevance Reaxys_biocatalysis model, leveraging a vast database of chemical reactions to predict feasible synthetic routes.
One-Step Synthesis Focus: Specifically designed for one-step synthesis, it provides concise and direct routes for your target compounds, streamlining the synthesis process.
Accurate Predictions: Utilizing the extensive PISTACHIO, BKMS_METABOLIC, PISTACHIO_RINGBREAKER, REAXYS, REAXYS_BIOCATALYSIS database, our tool offers high-accuracy predictions, reflecting the latest in chemical research and data.
Strategy Settings
Precursor scoring | Relevance Heuristic |
---|---|
Min. plausibility | 0.01 |
Model | Template_relevance |
Template Set | Pistachio/Bkms_metabolic/Pistachio_ringbreaker/Reaxys/Reaxys_biocatalysis |
Top-N result to add to graph | 6 |
Feasible Synthetic Routes
Haftungsausschluss und Informationen zu In-Vitro-Forschungsprodukten
Bitte beachten Sie, dass alle Artikel und Produktinformationen, die auf BenchChem präsentiert werden, ausschließlich zu Informationszwecken bestimmt sind. Die auf BenchChem zum Kauf angebotenen Produkte sind speziell für In-vitro-Studien konzipiert, die außerhalb lebender Organismen durchgeführt werden. In-vitro-Studien, abgeleitet von dem lateinischen Begriff "in Glas", beinhalten Experimente, die in kontrollierten Laborumgebungen unter Verwendung von Zellen oder Geweben durchgeführt werden. Es ist wichtig zu beachten, dass diese Produkte nicht als Arzneimittel oder Medikamente eingestuft sind und keine Zulassung der FDA für die Vorbeugung, Behandlung oder Heilung von medizinischen Zuständen, Beschwerden oder Krankheiten erhalten haben. Wir müssen betonen, dass jede Form der körperlichen Einführung dieser Produkte in Menschen oder Tiere gesetzlich strikt untersagt ist. Es ist unerlässlich, sich an diese Richtlinien zu halten, um die Einhaltung rechtlicher und ethischer Standards in Forschung und Experiment zu gewährleisten.