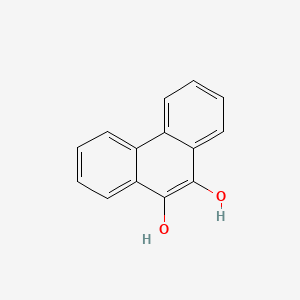
9,10-Dihydroxyphenanthrene
Übersicht
Beschreibung
9,10-Dihydroxyphenanthrene is an organic compound with the molecular formula C14H12O2 It is a derivative of phenanthrene, a polycyclic aromatic hydrocarbon This compound is characterized by the presence of two hydroxyl groups attached to the 9th and 10th positions of the phenanthrene ring system
Vorbereitungsmethoden
Synthetic Routes and Reaction Conditions
The synthesis of 9,10-Dihydroxyphenanthrene can be achieved through several methods. One common approach involves the oxidation of phenanthrene using strong oxidizing agents such as potassium permanganate or chromium trioxide. The reaction typically occurs under acidic conditions and requires careful control of temperature and reaction time to achieve the desired product .
Another method involves the photochemical reaction of 9,10-phenanthraquinone with glyoxals. This reaction produces monoesters of this compound along with the evolution of carbon monoxide .
Industrial Production Methods
Industrial production of this compound often involves large-scale oxidation processes using efficient and cost-effective oxidizing agents. The reaction conditions are optimized to maximize yield and minimize by-products. The product is then purified through crystallization or chromatography techniques to obtain high-purity this compound .
Analyse Chemischer Reaktionen
Types of Reactions
9,10-Dihydroxyphenanthrene undergoes various chemical reactions, including:
Oxidation: It can be further oxidized to form phenanthrenequinone.
Reduction: Reduction reactions can convert it back to phenanthrene.
Substitution: The hydroxyl groups can participate in substitution reactions to form ethers or esters.
Common Reagents and Conditions
Oxidation: Potassium permanganate, chromium trioxide, and other strong oxidizing agents under acidic conditions.
Reduction: Hydrogen gas in the presence of a palladium catalyst.
Substitution: Alkyl halides or acyl chlorides in the presence of a base.
Major Products Formed
Oxidation: Phenanthrenequinone.
Reduction: Phenanthrene.
Substitution: Ethers or esters depending on the substituent used.
Wissenschaftliche Forschungsanwendungen
Chemical Applications
Synthesis Precursor:
- 9,10-Dihydroxyphenanthrene serves as a precursor in the synthesis of various organic compounds, particularly in the development of polycyclic aromatic hydrocarbons and their derivatives.
Reagent in Photochemical Reactions:
- The compound is utilized as a reagent in photochemical reactions, enabling researchers to explore light-induced chemical transformations.
Biological Applications
Antimicrobial Properties:
- Studies have indicated that this compound exhibits antimicrobial activity, making it a candidate for developing new antimicrobial agents.
Anticancer Activity:
- Research has shown potential anticancer properties of this compound. For instance, analogs of related compounds have induced apoptosis in cancer cell lines, suggesting that this compound may also possess similar effects .
Mechanisms of Action:
- The compound's biological effects are believed to arise from its ability to generate reactive oxygen species (ROS), leading to cellular stress and apoptosis in targeted cells . This mechanism is particularly relevant in the context of cancer therapy.
Medical Applications
Therapeutic Potential:
- Ongoing research is investigating the therapeutic potential of this compound for treating various diseases, including its role as a possible inhibitor of viral proteins like SARS-CoV-2's main protease (3CLpro) . The structure-activity relationship studies have revealed that certain derivatives exhibit potent inhibitory activity against this viral target.
Industrial Applications
Dyes and Pigments:
- The compound is employed in the production of dyes and pigments due to its vibrant color properties and stability under various conditions.
Chemical Manufacturing:
- Beyond dyes, it finds use in manufacturing other industrial chemicals, contributing to various sectors including materials science and environmental chemistry.
Case Study 1: Cytotoxicity Mechanisms
A study explored the cytotoxic effects of this compound analogs on human cancer cell lines. It was found that these compounds can induce DNA fragmentation and apoptosis through ROS generation, highlighting their potential as anticancer agents .
Case Study 2: SARS-CoV-2 Inhibition
Research focused on the inhibition of SARS-CoV-2's main protease by derivatives of this compound demonstrated significant inhibitory effects with IC50 values around 1.55 μM. This positions the compound as a promising candidate for antiviral drug development .
Wirkmechanismus
The mechanism of action of 9,10-Dihydroxyphenanthrene involves its interaction with specific molecular targets and pathways. For example, in biological systems, it can undergo enzymatic oxidation to form reactive intermediates that interact with cellular components. These interactions can lead to various biological effects, including antimicrobial and anticancer activities .
Vergleich Mit ähnlichen Verbindungen
Similar Compounds
9,10-Phenanthrenediol: Similar structure but lacks the dihydroxy groups.
9,10-Dihydro-9,10-dihydroxyphenanthrene: A closely related compound with similar chemical properties.
Uniqueness
9,10-Dihydroxyphenanthrene is unique due to its specific hydroxylation pattern, which imparts distinct chemical reactivity and biological activity.
Biologische Aktivität
9,10-Dihydroxyphenanthrene (9,10-DHP) is a polycyclic aromatic hydrocarbon (PAH) derivative that has garnered attention for its diverse biological activities. This article explores its biological effects, mechanisms of action, and potential therapeutic applications based on recent research findings.
Chemical Structure and Properties
9,10-DHP is characterized by its two hydroxyl groups located at the 9 and 10 positions of the phenanthrene backbone. This structural modification significantly enhances its reactivity compared to its parent compound, phenanthrene.
Antiviral Properties
Recent studies have highlighted the potential of 9,10-DHP derivatives as inhibitors of the SARS-CoV-2 3CL protease, an essential enzyme for viral replication. Among various derivatives tested, compounds C1 and C2 exhibited potent inhibition with IC50 values of 1.55 ± 0.21 μM and 1.81 ± 0.17 μM, respectively. These compounds were shown to inhibit the protease in a mixed-inhibition manner, indicating their potential as therapeutic agents against COVID-19 .
Compound | IC50 (μM) | Inhibition Type |
---|---|---|
C1 | 1.55 ± 0.21 | Mixed |
C2 | 1.81 ± 0.17 | Mixed |
Cytotoxic Effects
9,10-DHP has also been implicated in cytotoxicity through mechanisms involving oxidative stress. It generates reactive oxygen species (ROS), which can lead to cellular damage and apoptosis in various cell lines. For instance, studies indicate that treatment with 9,10-PQ (a related quinone) leads to significant DNA fragmentation and apoptosis in human cells . The compound’s ability to induce oxidative stress is critical in understanding its role in toxicology and potential therapeutic applications.
Neuroinflammation
Research has demonstrated that 9,10-DHP exhibits antineuroinflammatory properties. In vitro studies using cell lines have shown that it can modulate inflammatory responses, potentially offering therapeutic avenues for neurodegenerative diseases .
The biological activity of 9,10-DHP is primarily mediated through:
- Oxidative Stress : The generation of ROS leads to cellular damage and apoptosis.
- Enzyme Inhibition : As noted in antiviral studies, specific derivatives inhibit key viral enzymes.
- Inflammatory Modulation : The compound's effects on inflammatory pathways suggest a role in managing neuroinflammation.
Antitumor Activity
In a study involving HCT-116 colon cancer cells and HL-60 leukemia cells, analogs of 9,10-PQ induced apoptosis effectively. The findings suggest that similar mechanisms may be applicable to 9,10-DHP derivatives .
Environmental Impact
Research indicates that compounds like 9,10-DHP can be found in environmental pollutants such as diesel exhaust particulates. Their presence raises concerns regarding human exposure and health implications due to their cytotoxic properties .
Q & A
Basic Research Questions
Q. How is 9,10-dihydroxyphenanthrene identified as a metabolite in microbial degradation studies?
To identify this compound, researchers typically use gas chromatography-mass spectrometry (GC-MS) after solvent extraction of microbial cultures. For example, in phenanthrene degradation studies with Coriolopsis byrsina strain APC5, GC-MS analysis detected this compound (retention time: 17.58 min) as a key intermediate metabolite . Enzyme activity assays (e.g., lignin peroxidase [LiP], manganese peroxidase [MnP], laccase [LAC]) are performed in parallel to correlate metabolite formation with enzymatic stages .
Q. What enzymatic systems are involved in the degradation of this compound?
Ligninolytic enzymes, particularly MnP, LiP, and LAC, drive the degradation. In fungal systems, MnP exhibits peak activity (20,147 U/L) during the initial phase (6–8 days), while LAC activity (2,629 U/L) dominates later stages, facilitating ring cleavage into metabolites like 2,2-diphenic acid and phthalic acid . Time-course enzyme assays (every 2 days) are critical to mapping activity profiles .
Q. How is this compound synthesized and purified for in vitro studies?
Synthesis involves microbial oxidation of phenanthrene via Pseudomonas stutzeri or fungal strains, followed by extraction under anoxic conditions (e.g., ethyl acetate/argon) to prevent auto-oxidation . Purification employs column chromatography or preparative HPLC. Structural validation is performed via NMR and high-resolution mass spectrometry (HRMS) .
Advanced Research Questions
Q. Why do certain dehydrogenases fail to catalyze this compound despite substrate similarities?
The PDDH dehydrogenase from Sphingomonas CHY-1 acts on dihydrodiols like benz[a]anthracene-1,2-dihydrodiol but not this compound due to steric hindrance from the hydroxyl group positions. Substrate specificity studies using UV/visible spectral shifts (260–400 nm) and NAD+ cofactor assays under argon reveal structural incompatibility . Comparative molecular docking can further elucidate active-site constraints .
Q. How can researchers resolve contradictions in reported enzyme roles across degradation pathways?
Discrepancies arise from differences in microbial strains, experimental conditions (e.g., aerobic vs. anoxic), and enzyme isoforms. For example, MnP dominates in C. byrsina , whereas bacterial systems rely on dioxygenases . Meta-analyses of time-resolved enzyme activity data (e.g., U/L trends over 8 days) and metabolite profiling (GC-MS) help reconcile pathway variations .
Q. What methodological challenges arise in detecting this compound auto-oxidation artifacts?
Auto-oxidation of dihydroxyphenanthrenes to o-quinones occurs rapidly in air-saturated buffers, confounding metabolite analysis. Researchers mitigate this by extracting metabolites under argon and using reducing agents (e.g., NADH) to stabilize intermediates. Spectrophotometric monitoring (e.g., 270 nm decay over 120 minutes) quantifies oxidation rates .
Q. How do in vitro and in vivo models differ in this compound metabolism?
In vitro systems (e.g., fungal cultures) prioritize enzyme-driven degradation, while in vivo models (e.g., rats, rabbits) involve phase I/II metabolism (e.g., glucuronidation). Historical studies in rabbits identified conjugated glucuronic acid derivatives of this compound, validated via hydrolysis and thin-layer chromatography (TLC) . Cross-species comparisons require LC-MS/MS to track conjugates .
Q. Experimental Design & Data Analysis
Q. How to optimize experimental timelines for studying this compound degradation kinetics?
Time-course experiments should sample every 48 hours for ≥8 days to capture enzyme activity peaks (e.g., MnP at day 6, LAC at day 8) . Parallel GC-MS profiling at days 0, 4, and 8 links metabolite accumulation to enzymatic phases. Statistical tools like ANOVA assess significance of degradation rates across replicates .
Q. What analytical strategies distinguish this compound from positional isomers?
High-performance liquid chromatography (HPLC) with photodiode array detection (PDA) separates isomers based on retention times and UV spectra (e.g., 9,10- vs. 3,4-dihydroxyphenanthrene). Confirmation via tandem MS/MS fragments (e.g., m/z 212 → 181) enhances specificity . Isotopic labeling (e.g., 13C) in tracer studies further resolves metabolic fates .
Q. How to address low yields in synthetic routes to this compound derivatives?
Current chemical synthesis methods yield ≤42% due to side reactions during epoxidation . Optimization involves catalyst screening (e.g., Sharpless asymmetric dihydroxylation) and solvent polarity adjustments. Fluorescence spectroscopy compares derived products (e.g., 9,10-phenanthrenequinone) to reference standards .
Eigenschaften
IUPAC Name |
phenanthrene-9,10-diol | |
---|---|---|
Source | PubChem | |
URL | https://pubchem.ncbi.nlm.nih.gov | |
Description | Data deposited in or computed by PubChem | |
InChI |
InChI=1S/C14H10O2/c15-13-11-7-3-1-5-9(11)10-6-2-4-8-12(10)14(13)16/h1-8,15-16H | |
Source | PubChem | |
URL | https://pubchem.ncbi.nlm.nih.gov | |
Description | Data deposited in or computed by PubChem | |
InChI Key |
ODUSUXJNDWKJKH-UHFFFAOYSA-N | |
Source | PubChem | |
URL | https://pubchem.ncbi.nlm.nih.gov | |
Description | Data deposited in or computed by PubChem | |
Canonical SMILES |
C1=CC=C2C(=C1)C3=CC=CC=C3C(=C2O)O | |
Source | PubChem | |
URL | https://pubchem.ncbi.nlm.nih.gov | |
Description | Data deposited in or computed by PubChem | |
Molecular Formula |
C14H10O2 | |
Source | PubChem | |
URL | https://pubchem.ncbi.nlm.nih.gov | |
Description | Data deposited in or computed by PubChem | |
DSSTOX Substance ID |
DTXSID20209159 | |
Record name | 9,10-Phenanthrenediol | |
Source | EPA DSSTox | |
URL | https://comptox.epa.gov/dashboard/DTXSID20209159 | |
Description | DSSTox provides a high quality public chemistry resource for supporting improved predictive toxicology. | |
Molecular Weight |
210.23 g/mol | |
Source | PubChem | |
URL | https://pubchem.ncbi.nlm.nih.gov | |
Description | Data deposited in or computed by PubChem | |
CAS No. |
604-84-2 | |
Record name | 9,10-Phenanthrenediol | |
Source | CAS Common Chemistry | |
URL | https://commonchemistry.cas.org/detail?cas_rn=604-84-2 | |
Description | CAS Common Chemistry is an open community resource for accessing chemical information. Nearly 500,000 chemical substances from CAS REGISTRY cover areas of community interest, including common and frequently regulated chemicals, and those relevant to high school and undergraduate chemistry classes. This chemical information, curated by our expert scientists, is provided in alignment with our mission as a division of the American Chemical Society. | |
Explanation | The data from CAS Common Chemistry is provided under a CC-BY-NC 4.0 license, unless otherwise stated. | |
Record name | 9,10-Phenanthrenediol | |
Source | ChemIDplus | |
URL | https://pubchem.ncbi.nlm.nih.gov/substance/?source=chemidplus&sourceid=0000604842 | |
Description | ChemIDplus is a free, web search system that provides access to the structure and nomenclature authority files used for the identification of chemical substances cited in National Library of Medicine (NLM) databases, including the TOXNET system. | |
Record name | 9,10-Phenanthrenediol | |
Source | EPA DSSTox | |
URL | https://comptox.epa.gov/dashboard/DTXSID20209159 | |
Description | DSSTox provides a high quality public chemistry resource for supporting improved predictive toxicology. | |
Retrosynthesis Analysis
AI-Powered Synthesis Planning: Our tool employs the Template_relevance Pistachio, Template_relevance Bkms_metabolic, Template_relevance Pistachio_ringbreaker, Template_relevance Reaxys, Template_relevance Reaxys_biocatalysis model, leveraging a vast database of chemical reactions to predict feasible synthetic routes.
One-Step Synthesis Focus: Specifically designed for one-step synthesis, it provides concise and direct routes for your target compounds, streamlining the synthesis process.
Accurate Predictions: Utilizing the extensive PISTACHIO, BKMS_METABOLIC, PISTACHIO_RINGBREAKER, REAXYS, REAXYS_BIOCATALYSIS database, our tool offers high-accuracy predictions, reflecting the latest in chemical research and data.
Strategy Settings
Precursor scoring | Relevance Heuristic |
---|---|
Min. plausibility | 0.01 |
Model | Template_relevance |
Template Set | Pistachio/Bkms_metabolic/Pistachio_ringbreaker/Reaxys/Reaxys_biocatalysis |
Top-N result to add to graph | 6 |
Feasible Synthetic Routes
Haftungsausschluss und Informationen zu In-Vitro-Forschungsprodukten
Bitte beachten Sie, dass alle Artikel und Produktinformationen, die auf BenchChem präsentiert werden, ausschließlich zu Informationszwecken bestimmt sind. Die auf BenchChem zum Kauf angebotenen Produkte sind speziell für In-vitro-Studien konzipiert, die außerhalb lebender Organismen durchgeführt werden. In-vitro-Studien, abgeleitet von dem lateinischen Begriff "in Glas", beinhalten Experimente, die in kontrollierten Laborumgebungen unter Verwendung von Zellen oder Geweben durchgeführt werden. Es ist wichtig zu beachten, dass diese Produkte nicht als Arzneimittel oder Medikamente eingestuft sind und keine Zulassung der FDA für die Vorbeugung, Behandlung oder Heilung von medizinischen Zuständen, Beschwerden oder Krankheiten erhalten haben. Wir müssen betonen, dass jede Form der körperlichen Einführung dieser Produkte in Menschen oder Tiere gesetzlich strikt untersagt ist. Es ist unerlässlich, sich an diese Richtlinien zu halten, um die Einhaltung rechtlicher und ethischer Standards in Forschung und Experiment zu gewährleisten.