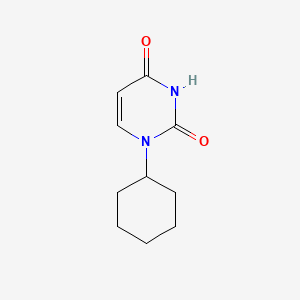
1-Cyclohexyluracil
Übersicht
Beschreibung
1-Cyclohexyluracil is a derivative of uracil, one of the five canonical nucleobases that constitute the genetic alphabet in DNA and RNA. This compound features a cyclohexyl group covalently bonded to the nitrogen atom at the first position of the uracil ring. The modification of uracil with a cyclohexyl group alters its chemical and physical properties, making it a subject of interest in various scientific research fields .
Wissenschaftliche Forschungsanwendungen
1-Cyclohexyluracil has several scientific research applications:
Biology: The compound’s structural similarity to uracil makes it useful in studying nucleic acid interactions and enzyme-substrate specificity.
Industry: Used in the synthesis of specialized materials and as a precursor in various chemical processes.
Wirkmechanismus
Target of Action
1-Cyclohexyluracil (1CHU) is a derivative of uracil, a component of RNA . The primary target of 1CHU is the bright state, a state of high energy in the molecule .
Mode of Action
Upon UV excitation, 1CHU populates the bright state . From this state, two likely deactivation pathways can occur . The first pathway (Route 1) is similar to the relaxation mechanism observed for uracil, where there is an internal conversion to the ground state through an ethylenic-like conical intersection . The second pathway (Route 2) involves a direct population transfer from the bright state to the triplet state via an intersystem crossing process .
Biochemical Pathways
The biochemical pathways of 1CHU involve photophysical relaxation mechanisms . These mechanisms are crucial for maintaining the photostability of the nucleobases in DNA and RNA, minimizing the effects of undesirable photoexcited chemical reactions .
Pharmacokinetics
Its photophysical properties have been investigated in both vacuum and water environments . These studies suggest that the environment can significantly influence the deactivation pathways and the lifetime of the bright state .
Result of Action
The result of 1CHU’s action is the ultrafast deactivation of the bright state . Most of the electronic population initially on the bright state returns to the ground state, following the same ultrafast deactivation mechanism observed in uracil (Route 1), while a smaller percentage goes to the triplet manifold .
Action Environment
The action of 1CHU is influenced by the environment. In both vacuum and water environments, the primary photophysical event is the population of the bright state . The presence of a minimum on the potential energy hypersurface in water can help to understand why experimentally it is noticed suppression of the triplet states population in polar protic solvent .
Biochemische Analyse
Biochemical Properties
1-Cyclohexyluracil plays a significant role in various biochemical reactions. It interacts with several enzymes, proteins, and other biomolecules. For instance, it has been observed to interact with enzymes involved in nucleotide metabolism, such as thymidine phosphorylase. The nature of these interactions often involves binding to the active site of the enzyme, thereby influencing its activity. Additionally, this compound can form hydrogen bonds and hydrophobic interactions with proteins, affecting their conformation and function .
Cellular Effects
This compound exerts various effects on different types of cells and cellular processes. It has been shown to influence cell signaling pathways, gene expression, and cellular metabolism. For example, this compound can modulate the activity of transcription factors, leading to changes in gene expression profiles. It also affects cellular metabolism by altering the activity of key metabolic enzymes, thereby impacting the overall metabolic flux within the cell .
Molecular Mechanism
The molecular mechanism of action of this compound involves several key processes. At the molecular level, it binds to specific biomolecules, such as enzymes and receptors, through hydrogen bonding and hydrophobic interactions. This binding can result in either inhibition or activation of the target enzyme, depending on the context. Additionally, this compound can influence gene expression by interacting with transcription factors and other regulatory proteins, leading to changes in the transcriptional activity of specific genes .
Temporal Effects in Laboratory Settings
In laboratory settings, the effects of this compound can change over time. The compound is relatively stable at room temperature but may degrade under certain conditions, such as exposure to light or extreme pH. Long-term studies have shown that this compound can have sustained effects on cellular function, including prolonged changes in gene expression and metabolic activity. These temporal effects are crucial for understanding the long-term implications of using this compound in research and therapeutic applications .
Dosage Effects in Animal Models
The effects of this compound vary with different dosages in animal models. At low doses, the compound may have minimal impact on cellular function, while higher doses can lead to significant changes in gene expression and metabolic activity. Toxic or adverse effects have been observed at very high doses, including cellular toxicity and disruption of normal cellular processes. These dosage-dependent effects are important for determining the safe and effective use of this compound in research and potential therapeutic applications .
Metabolic Pathways
This compound is involved in several metabolic pathways, particularly those related to nucleotide metabolism. It interacts with enzymes such as thymidine phosphorylase and dihydropyrimidine dehydrogenase, influencing the breakdown and synthesis of nucleotides. These interactions can affect the overall metabolic flux and levels of various metabolites within the cell, highlighting the compound’s role in cellular metabolism .
Transport and Distribution
Within cells and tissues, this compound is transported and distributed through various mechanisms. It can be taken up by cells via specific transporters or passive diffusion. Once inside the cell, it may bind to transport proteins that facilitate its distribution to different cellular compartments. The localization and accumulation of this compound within specific tissues can influence its overall activity and function .
Subcellular Localization
The subcellular localization of this compound is crucial for its activity and function. It can be directed to specific compartments or organelles through targeting signals or post-translational modifications. For example, this compound may localize to the nucleus, where it can interact with transcription factors and influence gene expression. Alternatively, it may be found in the cytoplasm, where it can affect metabolic enzymes and other cellular processes .
Vorbereitungsmethoden
Synthetic Routes and Reaction Conditions
1-Cyclohexyluracil can be synthesized through the alkylation of uracil. The process typically involves the reaction of uracil with cyclohexyl bromide in the presence of a base such as potassium carbonate. The reaction is carried out in a polar aprotic solvent like dimethylformamide at elevated temperatures to facilitate the nucleophilic substitution reaction .
Industrial Production Methods
While specific industrial production methods for this compound are not extensively documented, the general approach would involve scaling up the laboratory synthesis process. This would include optimizing reaction conditions, such as temperature and solvent choice, to maximize yield and purity. Continuous flow reactors and automated synthesis platforms could be employed to enhance efficiency and reproducibility.
Analyse Chemischer Reaktionen
Types of Reactions
1-Cyclohexyluracil undergoes various chemical reactions, including:
Oxidation: The compound can be oxidized to form corresponding uracil derivatives with altered functional groups.
Reduction: Reduction reactions can modify the cyclohexyl group or the uracil ring itself.
Substitution: Nucleophilic substitution reactions can introduce different substituents at various positions on the uracil ring.
Common Reagents and Conditions
Oxidation: Common oxidizing agents include potassium permanganate and chromium trioxide.
Reduction: Reducing agents such as sodium borohydride or lithium aluminum hydride are typically used.
Substitution: Bases like potassium carbonate and solvents like dimethylformamide are commonly employed.
Major Products Formed
The major products formed from these reactions depend on the specific reagents and conditions used. For example, oxidation may yield hydroxylated or ketone derivatives, while substitution reactions can introduce various alkyl or aryl groups.
Vergleich Mit ähnlichen Verbindungen
Similar Compounds
Uracil: The parent compound, which lacks the cyclohexyl group.
Thymine: Another pyrimidine nucleobase with a methyl group at the fifth position.
Cytosine: A pyrimidine nucleobase with an amino group at the fourth position.
Uniqueness
1-Cyclohexyluracil is unique due to the presence of the cyclohexyl group, which significantly alters its chemical and physical properties compared to other nucleobases. This modification enhances its photophysical stability and makes it a valuable tool in studying nucleobase analogues .
Eigenschaften
IUPAC Name |
1-cyclohexylpyrimidine-2,4-dione | |
---|---|---|
Source | PubChem | |
URL | https://pubchem.ncbi.nlm.nih.gov | |
Description | Data deposited in or computed by PubChem | |
InChI |
InChI=1S/C10H14N2O2/c13-9-6-7-12(10(14)11-9)8-4-2-1-3-5-8/h6-8H,1-5H2,(H,11,13,14) | |
Source | PubChem | |
URL | https://pubchem.ncbi.nlm.nih.gov | |
Description | Data deposited in or computed by PubChem | |
InChI Key |
SYRKUUKGYSRJPB-UHFFFAOYSA-N | |
Source | PubChem | |
URL | https://pubchem.ncbi.nlm.nih.gov | |
Description | Data deposited in or computed by PubChem | |
Canonical SMILES |
C1CCC(CC1)N2C=CC(=O)NC2=O | |
Source | PubChem | |
URL | https://pubchem.ncbi.nlm.nih.gov | |
Description | Data deposited in or computed by PubChem | |
Molecular Formula |
C10H14N2O2 | |
Source | PubChem | |
URL | https://pubchem.ncbi.nlm.nih.gov | |
Description | Data deposited in or computed by PubChem | |
DSSTOX Substance ID |
DTXSID80221397 | |
Record name | 1-Cyclohexyluracil | |
Source | EPA DSSTox | |
URL | https://comptox.epa.gov/dashboard/DTXSID80221397 | |
Description | DSSTox provides a high quality public chemistry resource for supporting improved predictive toxicology. | |
Molecular Weight |
194.23 g/mol | |
Source | PubChem | |
URL | https://pubchem.ncbi.nlm.nih.gov | |
Description | Data deposited in or computed by PubChem | |
CAS No. |
712-43-6 | |
Record name | 1-Cyclohexyluracil | |
Source | ChemIDplus | |
URL | https://pubchem.ncbi.nlm.nih.gov/substance/?source=chemidplus&sourceid=0000712436 | |
Description | ChemIDplus is a free, web search system that provides access to the structure and nomenclature authority files used for the identification of chemical substances cited in National Library of Medicine (NLM) databases, including the TOXNET system. | |
Record name | 712-43-6 | |
Source | DTP/NCI | |
URL | https://dtp.cancer.gov/dtpstandard/servlet/dwindex?searchtype=NSC&outputformat=html&searchlist=76947 | |
Description | The NCI Development Therapeutics Program (DTP) provides services and resources to the academic and private-sector research communities worldwide to facilitate the discovery and development of new cancer therapeutic agents. | |
Explanation | Unless otherwise indicated, all text within NCI products is free of copyright and may be reused without our permission. Credit the National Cancer Institute as the source. | |
Record name | 1-Cyclohexyluracil | |
Source | EPA DSSTox | |
URL | https://comptox.epa.gov/dashboard/DTXSID80221397 | |
Description | DSSTox provides a high quality public chemistry resource for supporting improved predictive toxicology. | |
Retrosynthesis Analysis
AI-Powered Synthesis Planning: Our tool employs the Template_relevance Pistachio, Template_relevance Bkms_metabolic, Template_relevance Pistachio_ringbreaker, Template_relevance Reaxys, Template_relevance Reaxys_biocatalysis model, leveraging a vast database of chemical reactions to predict feasible synthetic routes.
One-Step Synthesis Focus: Specifically designed for one-step synthesis, it provides concise and direct routes for your target compounds, streamlining the synthesis process.
Accurate Predictions: Utilizing the extensive PISTACHIO, BKMS_METABOLIC, PISTACHIO_RINGBREAKER, REAXYS, REAXYS_BIOCATALYSIS database, our tool offers high-accuracy predictions, reflecting the latest in chemical research and data.
Strategy Settings
Precursor scoring | Relevance Heuristic |
---|---|
Min. plausibility | 0.01 |
Model | Template_relevance |
Template Set | Pistachio/Bkms_metabolic/Pistachio_ringbreaker/Reaxys/Reaxys_biocatalysis |
Top-N result to add to graph | 6 |
Feasible Synthetic Routes
Q1: What makes 1-Cyclohexyluracil a valuable tool for studying nucleic acid interactions?
A1: this compound mimics the hydrogen bonding behavior of uracil, a core component of RNA. Studies demonstrate that 1CHU forms hydrogen-bonded complexes with adenine derivatives, allowing researchers to explore the specific interactions involved in base pairing. [, , , , , , ]
Q2: What is the significance of studying the hydrogen bonding behavior of 1CHU in solutions?
A3: Understanding the self-aggregation properties of 1CHU in solutions like deuterochloroform (CDCl3) provides insights into the forces driving nucleic acid interactions. Infrared (IR) spectroscopic studies show that 1CHU forms dimers in CDCl3, with two coexisting isomeric structures having similar abundance. This emphasizes the role of non-covalent interactions in shaping nucleic acid structures. [, ]
Q3: How does 1CHU's structure contribute to its aggregation behavior?
A4: While 1CHU forms dimers in solution, research suggests that it does not readily form higher-order aggregates beyond the dimer stage. This contrasts with adenine and cytosine derivatives, indicating that the specific structural features of 1CHU influence its self-association behavior. []
Q4: Beyond its interactions with adenine, has 1CHU been studied in the context of other molecules?
A5: Yes, 1CHU has been investigated for its interactions with molecules beyond adenine derivatives. For example, studies have explored its hydrogen bonding with caffeine and theophylline in chloroform solution. Notably, theophylline demonstrates stronger association with 9-ethyladenine compared to caffeine, suggesting potential implications for their respective interactions with nucleic acids. []
Q5: How does the presence of a solvent affect the photophysical properties of 1CHU?
A6: Solvent polarity significantly impacts the excited-state dynamics of 1CHU. Femtosecond transient absorption spectroscopy reveals that after UV excitation, the initial excited state population splits: approximately 60% decays to the ground state, while the remainder transitions to a longer-lived singlet dark state. This dark state's lifetime varies from picoseconds in water to nanoseconds in aprotic solvents. [, ]
Q6: What insights do computational chemistry studies provide into the photophysical behavior of 1CHU?
A7: Quantum chemical calculations, particularly those employing the Multi-State CASPT2 method, have been crucial in elucidating the deactivation pathways of 1CHU. These studies suggest that the primary photophysical event is the population of the S11(ππ) bright state, followed by either internal conversion to the ground state or intersystem crossing to a triplet state. The presence of water can influence these pathways, as evidenced by a minimum observed on the S11(ππ) potential energy surface in aqueous environments. []
Q7: Have researchers used computational methods to simulate the vibrational properties of 1CHU?
A8: Yes, hybrid Car-Parrinello QM/MM molecular dynamics simulations have been employed to analyze the hydrogen bond dynamics of an adenine:uracil base pair, using 1CHU and 9-ethyl-8-phenyladenine in deuterochloroform solution. These simulations successfully reproduced the infrared absorption spectrum of the system, validating the accuracy of the computational approach in studying 1CHU's vibrational properties. []
Haftungsausschluss und Informationen zu In-Vitro-Forschungsprodukten
Bitte beachten Sie, dass alle Artikel und Produktinformationen, die auf BenchChem präsentiert werden, ausschließlich zu Informationszwecken bestimmt sind. Die auf BenchChem zum Kauf angebotenen Produkte sind speziell für In-vitro-Studien konzipiert, die außerhalb lebender Organismen durchgeführt werden. In-vitro-Studien, abgeleitet von dem lateinischen Begriff "in Glas", beinhalten Experimente, die in kontrollierten Laborumgebungen unter Verwendung von Zellen oder Geweben durchgeführt werden. Es ist wichtig zu beachten, dass diese Produkte nicht als Arzneimittel oder Medikamente eingestuft sind und keine Zulassung der FDA für die Vorbeugung, Behandlung oder Heilung von medizinischen Zuständen, Beschwerden oder Krankheiten erhalten haben. Wir müssen betonen, dass jede Form der körperlichen Einführung dieser Produkte in Menschen oder Tiere gesetzlich strikt untersagt ist. Es ist unerlässlich, sich an diese Richtlinien zu halten, um die Einhaltung rechtlicher und ethischer Standards in Forschung und Experiment zu gewährleisten.