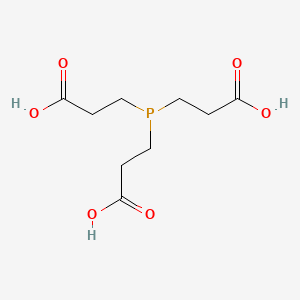
Tris(2-carboxyethyl)phosphine
Übersicht
Beschreibung
Vorbereitungsmethoden
Tris(2-carboxyethyl)phosphine can be synthesized through the acid hydrolysis of tris(cyanoethyl)phosphine in refluxing aqueous hydrochloric acid . The reaction involves the following steps:
Alkylation Reaction: Tetrakisphosphonium chloride undergoes an alkylation reaction with a suitable compound in the presence of an organic solvent at a temperature range of 30-60°C.
Hydrolysis Reaction: The resulting compound from the alkylation reaction is then subjected to hydrolysis to yield this compound hydrochloride.
Analyse Chemischer Reaktionen
Reduction Reactions
Cleavage of Disulfide Bonds: TCEP's primary chemical reaction involves the reduction of disulfide bonds . The phosphorus atom in TCEP attacks one of the sulfur atoms in the S-S bond, leading to the formation of a thioalkoxyphosphonium cation and a sulfhydryl anion. Subsequent hydrolysis releases the second sulfhydryl molecule and forms phosphine oxide .
The general mechanism can be represented as :
-
Cleavage of the S-S bond.
-
Oxidation of the phosphine and release of sulfhydryl molecules.
Optimal Conditions: While TCEP reactions are often performed at room temperature, research indicates that conducting the reaction on ice can eliminate interference peaks in certain analyses, particularly with mouse serum samples . Reduction is largely completed within 5 minutes, reaching a plateau after 30 minutes on ice . Effective reducing pH is broad, with TCEP being more effective than DTT at pH < 8.0 .
Reaction with DTNB
TCEP reacts with 5,5'-dithiobis(2-nitrobenzoic acid) (DTNB), also known as Ellman’s reagent, which is used for quantitative determination of TCEP concentration . TCEP reduces DTNB rapidly and stoichiometrically, generating two equivalents of 2-nitro-5-thiobenzoate (NTB). The concentration of NTB can be measured spectrophotometrically, allowing for the determination of TCEP concentration .
NTB, in its anionic form, exhibits a molar extinction coefficient of 14,150 M cm at 412 nm . This method is sensitive enough to detect TCEP concentrations in the micromolar range and is useful for monitoring TCEP stability and oxidation under various conditions .
Reaction with NAD+
TCEP can react non-enzymatically with NAD, forming a covalent adduct. This reaction involves the phosphorus atom of TCEP and the C4 atom of the nicotinamide ring of NAD .
Implications: This finding suggests caution when using TCEP in experimental protocols involving NAD(P), as the resulting adduct can potentially inhibit enzymes .
Side Reactions
While TCEP is generally selective towards disulfide bonds, side reactions can occur under certain conditions . TCEP-related cleavage has been observed to fracture cysteine residues in proteins, leading to heterogeneous peptides .
Products: The peptide products include those with a primary amine and a carboxylic acid as termini, as well as amidated or formamidated carboxy termini, or formylated or glyoxylic amino termini . The formation of formamidated carboxy termini and glyoxylic amino termini involves breaking carbon-carbon bonds in cysteine, which is an unexpected reaction .
Interaction with Metal Complexes
TCEP is used as a ligand in metal complexes, such as those with platinum(II) and palladium(II) . These complexes have shown potential in biomedical applications, including anticancer activity .
Platinum(II) Complexes: The complex cis-[PtCl(TCEP)] exhibits cytotoxic activity against cisplatin-resistant tumor cells . It induces a caspase-dependent path of apoptosis, stemming from the lowering of Bcl-X protein’s expression and the increase in pro-apoptotic BAX protein . This complex can halt cell cycle progression in the G2/M phase in canine osteosarcoma and human cancer cell lines .
Palladium(II) Complexes: Palladium(II) complexes with TCEP, such as trans-[PdCl(TCEP)] (PdTCEP), also demonstrate antitumor activity against various cancer cell lines .
TCEP Reduction Optimization in Mouse Serum Samples
Optimization of TCEP reduction conditions in mouse serum samples revealed that conducting the TCEP reaction on ice, instead of at room temperature, eliminates interference peaks on chromatograms .
Table 1: Recovery of Thiols with TCEP Reduction on Ice
Thiols | Added [μM] | Measured (mean ± SD) [μM] | RSD [%] | Recovery [%] |
---|---|---|---|---|
Hcy | 0 | 25.8 ± 0.2 | 0.8 | - |
15 | 39.8 ± 0.2 | 0.6 | 93 | |
30 | 54.1 ± 0.2 | 0.4 | 94 | |
60 | 82.9 ± 0.2 | 0.2 | 95 | |
Cys | 0 | 178 ± 1 | 0.5 | - |
75 | 241 ± 2 | 0.8 | 84 | |
150 | 306 ± 1 | 0.3 | 85 | |
300 | 435 ± 1 | 0.2 | 86 | |
CysGly | 0 | 2.00 ± 0.01 | 0.7 | - |
1.5 | 3.31 ± 0.02 | 0.5 | 87 | |
3 | 4.64 ± 0.02 | 0.5 | 88 | |
6 | 7.31 ± 0.02 | 0.3 | 88 | |
GSH | 0 | 31.7 ± 0.4 | 1.4 | - |
15 | 45.1 ± 0.3 | 0.7 | 89 | |
30 | 58.8 ± 0.3 | 0.6 | 90 | |
60 | 88.2 ± 0.5 | 0.5 | 94 | |
γGluCys | 0 | 8.6 ± 0.2 | 2.3 | - |
7.5 | 15.6 ± 0.2 | 1.0 | 93 | |
15 | 22.6 ± 0.2 | 0.9 | 93 | |
30 | 37.6 ± 0.2 | 0.5 | 97 |
Table 2: Recovery of Thiols with TCEP Reduction at Room Temperature
Thiols | Added [μM] | Measured (mean ± SD) [μM] | RSD [%] | Recovery [%] |
---|---|---|---|---|
Hcy | 0 | 25.9 ± 0.4 | 1.5 | - |
15 | 39.6 ± 0.7 | 1.8 | 91 | |
30 | 53.7 ± 0.8 | 1.5 | 93 | |
60 | 81.7 ± 1.3 | 1.6 | 93 | |
Cys | 0 | 179 ± 3 | 1.8 | - |
75 | 241 ± 5 | 2.1 | 83 | |
150 | 304 ± 6 | 2.0 | 83 | |
300 | 431 ± 9 | 2.0 | 84 | |
CysGly | 0 | 2.01 ± 0.06 | 2.8 | - |
1.5 | 3.27 ± 0.08 | 2.6 | 84 | |
3 | 4.58 ± 0.09 | 2.0 | 86 | |
6 | 7.21 ± 0.17 | 2.4 | 87 | |
GSH | 0 | 31.5 ± 1.0 | 3.0 | - |
15 | 44.3 ± 1.2 | 2.7 | 85 | |
30 | 57.7 ± 1.2 | 2.1 | 87 | |
60 | 85.8 ± 1.8 | 2.1 | 90 | |
γGluCys | 0 | 8.3 ± 0.2 | 2.0 | - |
7.5 | 14.9 ± 0.3 | 2.0 | 88 | |
15 | 21.8 ± 0.4 | 1.8 | 90 | |
30 | 36.2 ± 0.9 | 2.6 | 93 |
Optimal Reaction Time: Reduction is largely completed after 5 min both at room temperature and on ice. After 30 min of reaction, a plateau was reached, indicating this time was sufficient .
Wissenschaftliche Forschungsanwendungen
Protein Chemistry
Reducing Disulfide Bonds
- TCEP is primarily used to break disulfide bonds within proteins, facilitating the study of protein structure and function. It allows for the complete reduction of disulfides in less than five minutes at room temperature, making it an efficient choice for protein denaturation prior to techniques such as gel electrophoresis .
Comparison of Reducing Agents
Property | TCEP | DTT | β-Mercaptoethanol |
---|---|---|---|
Odor | Odorless | Strong odor | Strong odor |
Stability | High | Low | Low |
Solubility in Water | High | Moderate | Moderate |
pH Range | Broad | Limited | Limited |
Drug Development
Anticancer Applications
- Recent studies have explored TCEP's role in enhancing the efficacy of platinum-based anticancer drugs. For instance, a platinum(II) complex with TCEP demonstrated superior cytotoxicity against canine lymphoma and leukemia cell lines compared to cisplatin. The complex induced apoptosis more effectively than traditional treatments .
Case Study: Platinum(II)-TCEP Complex
- In vitro studies revealed that the complex cis-[PtCl₂(TCEP)₂] exhibited higher cytotoxic activity than cisplatin, with nearly complete cell death observed at certain concentrations. This suggests potential for developing more effective cancer therapies utilizing TCEP derivatives .
Nanotechnology and Drug Delivery
Liposomal Formulations
- TCEP has been incorporated into liposomal drug delivery systems, which are designed to improve the bioavailability and targeting of therapeutic agents. Liposomes encapsulating drugs can enhance their stability and reduce side effects while improving delivery efficiency to tumor sites .
Advantages of Liposomal Systems
Feature | Benefit |
---|---|
Biocompatibility | Reduced toxicity |
Targeted Delivery | Increased efficacy at tumor sites |
Encapsulation | Protection from degradation |
Biochemical Assays
Role in Enzymatic Reactions
- TCEP is utilized in various biochemical assays where it prevents the formation of disulfide bonds during reactions involving cysteine residues. This is particularly important when labeling proteins with maleimides, as TCEP minimizes unwanted side reactions .
Impact on Assay Results
Wirkmechanismus
The mechanism of action of tris(2-carboxyethyl)phosphine involves the reduction of disulfide bonds in proteins and peptides. The phosphorus atom in this compound attacks one sulfur atom along the disulfide bond, forming a thioalkoxyphosphonium cation and a sulfhydryl anion . This is followed by rapid hydrolysis, releasing the second sulfhydryl molecule and forming phosphine oxide . This mechanism ensures the effective cleavage of disulfide bonds, making this compound a powerful reducing agent.
Vergleich Mit ähnlichen Verbindungen
Tris(2-carboxyethyl)phosphine is often compared with other reducing agents such as dithiothreitol and β-mercaptoethanol. Here are some key differences:
Odor: Unlike dithiothreitol and β-mercaptoethanol, this compound is odorless, making it more user-friendly.
Stability: This compound is more stable in aqueous solutions and less prone to oxidation compared to dithiothreitol.
Reactivity: It is a more powerful and irreversible reducing agent, ensuring complete reduction of disulfide bonds.
Hydrophilicity: This compound is more hydrophilic, which enhances its solubility and effectiveness in aqueous environments.
Similar compounds include:
- Dithiothreitol (DTT)
- β-Mercaptoethanol
- 2-Mercaptoethylamine
These compounds share similar reducing properties but differ in terms of odor, stability, and reactivity.
Biologische Aktivität
Tris(2-carboxyethyl)phosphine (TCEP) is a phosphine compound widely recognized for its role as a reducing agent in biochemical applications. Its unique properties have led to diverse biological activities, particularly in the fields of cancer research, enzyme activity modulation, and RNA stability. This article delves into the biological activity of TCEP, supported by research findings, data tables, and case studies.
Overview of TCEP
TCEP is commonly used in biochemistry due to its ability to reduce disulfide bonds in proteins without forming reactive byproducts. Unlike other reducing agents such as dithiothreitol (DTT), TCEP remains stable under various conditions and does not interfere with spectroscopic measurements in the UV range .
1. Anticancer Activity
Recent studies have highlighted the potential of TCEP in enhancing the efficacy of platinum-based anticancer drugs. A notable study investigated the complex of platinum(II) with TCEP (cis-[PtCl2(TCEP)2]), which demonstrated significant cytotoxicity against canine lymphoma and leukemia cell lines. The results indicated that this complex exhibited a higher cytotoxic effect compared to cisplatin, particularly at lower concentrations .
Key Findings:
- Cell Viability Assays: Pt-TCEP reduced cell viability significantly more than cisplatin across various concentrations.
- Mechanism of Action: The cytotoxicity was associated with apoptosis, as evidenced by flow cytometry analyses using Annexin V staining.
Cell Line | Viability (%) at 2.5 µM Pt-TCEP | Viability (%) at 15 µM CDDP |
---|---|---|
CLBL-1 | 5.33 ± 2.21 | Not statistically different from control |
GL-1 | 1.68 ± 0.47 | Not statistically different from control |
CL-1 | 26.50 ± 6.1 | 67.13 ± 1.58 |
2. Enzyme Activity Modulation
TCEP has been shown to influence enzyme activities by maintaining thiol groups in their reduced state, thereby stabilizing protein structures necessary for enzymatic function. For instance, it has been reported that TCEP can enhance the stability and activity of certain ribozymes by effectively preventing the formation of disulfide bonds that could lead to inactive conformations .
Case Study: RNA Stability
In experiments assessing RNA stability, TCEP was found to significantly increase the half-life of RNA at elevated temperatures compared to DTT and other reducing agents. This enhancement is crucial for applications involving RNA manipulation and analysis in molecular biology.
Condition | Half-Life Increase (TCEP vs DTT) |
---|---|
50 °C | More than double |
70 °C | More than double |
3. Interaction with Biological Cofactors
Research has also indicated that TCEP can interact with biological cofactors like NAD(P)+, potentially leading to their inactivation under certain conditions . This interaction raises caution regarding its use in assays involving these cofactors, as it may yield misleading results if not properly controlled.
Q & A
Basic Research Questions
Q. How does TCEP compare to dithiothreitol (DTT) in reducing disulfide bonds in protein research?
TCEP is superior to DTT due to its stability across a broader pH range (pH 2–9), resistance to air oxidation, and lack of disulfide exchange byproducts. Unlike DTT, TCEP does not form mixed disulfides with free thiols, simplifying downstream analyses. For example, TCEP is effective at 5–10 mM concentrations in SDS-PAGE sample buffers to fully reduce proteins without interfering with electrophoretic mobility . Additionally, TCEP’s non-thiol nature avoids artifacts in mass spectrometry (MS) workflows .
Q. What is the optimal concentration of TCEP for reducing disulfide bonds in proteins during SDS-PAGE?
The standard concentration ranges from 5–10 mM in denaturing buffers (e.g., 30 mM Tris-HCl, pH 7.8, with 50 mM NaCl and 10 mM MgCl₂). However, this should be adjusted based on the protein’s thiol content and buffer composition. For instance, in cytochrome c reduction protocols, 10 mM TCEP in 20 mM Tris-HCl (pH 7.0) with 500 mM sucrose ensures complete reduction while preserving protein stability .
Q. How does TCEP affect downstream applications like mass spectrometry?
TCEP minimizes interference in matrix-assisted laser desorption/ionization (MALDI) and electrospray ionization (ESI) MS by avoiding adduct formation. For example, TCEP is compatible with in-gel digestion workflows, where it reduces disulfides without generating background noise, unlike β-mercaptoethanol . In Co-IP-MS, TCEP cleaves cross-links while maintaining protein integrity, enabling accurate interaction mapping .
Advanced Research Questions
Q. How can researchers resolve contradictions in TCEP’s effectiveness under varying buffer conditions?
Contradictions often arise from interactions between TCEP and metal ions (e.g., Mg²⁺ or Zn²⁺) or pH-dependent solubility. For example:
- In CRISPR-Cas12a biosensors, TCEP (0.5–1 mM) is used in Tris-HCl (pH 7.8) with NaCl and MgCl₂, but higher Mg²⁺ concentrations (>20 mM) can chelate TCEP, reducing its efficacy. This requires empirical validation using Ellman’s assay to quantify free thiols .
- In alkaline conditions (pH >9), TCEP’s solubility decreases, necessitating buffer optimization or alternative reductants like tris(3-hydroxypropyl)phosphine (THPP) .
Q. What strategies optimize TCEP use in crosslinking studies without disrupting protein-protein interactions?
To preserve native interactions:
- Use lower TCEP concentrations (1–2 mM) during immunoprecipitation (IP) or affinity purification.
- Validate reduction efficiency via Western blotting or thiol-specific fluorophores (e.g., monobromobimane).
- For crosslinking reversal, incubate TCEP (10 mM) at 37°C for 1 hour in 50 mM Tris-HCl (pH 7.5) to cleave disulfide-linked complexes while retaining non-covalent interactions .
Q. How can researchers evaluate TCEP’s stability in long-term experiments?
TCEP decomposes slowly in aqueous solutions (t₁/₂ ~7 days at 25°C), but degradation accelerates in acidic or metal-rich buffers. Stability can be monitored via:
- NMR spectroscopy : Track the phosphine peak (δ ~20 ppm) disappearance.
- HPLC-MS : Detect oxidation byproducts like tris(2-carboxyethyl)phosphine oxide (TCEPO).
- Practical tip : Prepare fresh TCEP solutions daily or use stabilized formulations (e.g., 0.5 M HCl stock stored at -20°C) .
Q. How do researchers address discrepancies in TCEP’s reactivity with metal ions in catalytic assays?
TCEP can reduce metal ions (e.g., Cu²⁺ or Fe³⁺), altering catalytic activity. To mitigate this:
- Pre-treat buffers with chelators (e.g., EDTA) to sequester metals.
- Use alternative reductants in metal-dependent systems (e.g., ascorbate for Cu-catalyzed click chemistry).
- Validate via control experiments comparing reaction yields with/without TCEP .
Q. Methodological Tables
Table 1: TCEP vs. DTT in Common Applications
Parameter | TCEP | DTT |
---|---|---|
Effective pH range | 2–9 | 7–8.5 |
Air oxidation resistance | High | Low |
Interference in MS | Minimal | Adduct formation |
Typical concentration | 5–10 mM | 5–20 mM |
Table 2: TCEP Stability in Buffers
Buffer Condition | Half-life (25°C) | Recommendation |
---|---|---|
Neutral pH (7.0) | ~7 days | Prepare weekly, store at 4°C |
Acidic (pH 4.0) | <1 day | Use immediately |
Metal-rich (10 mM Mg²⁺) | <12 hours | Add EDTA (5 mM) |
Eigenschaften
IUPAC Name |
3-[bis(2-carboxyethyl)phosphanyl]propanoic acid | |
---|---|---|
Source | PubChem | |
URL | https://pubchem.ncbi.nlm.nih.gov | |
Description | Data deposited in or computed by PubChem | |
InChI |
InChI=1S/C9H15O6P/c10-7(11)1-4-16(5-2-8(12)13)6-3-9(14)15/h1-6H2,(H,10,11)(H,12,13)(H,14,15) | |
Source | PubChem | |
URL | https://pubchem.ncbi.nlm.nih.gov | |
Description | Data deposited in or computed by PubChem | |
InChI Key |
PZBFGYYEXUXCOF-UHFFFAOYSA-N | |
Source | PubChem | |
URL | https://pubchem.ncbi.nlm.nih.gov | |
Description | Data deposited in or computed by PubChem | |
Canonical SMILES |
C(CP(CCC(=O)O)CCC(=O)O)C(=O)O | |
Source | PubChem | |
URL | https://pubchem.ncbi.nlm.nih.gov | |
Description | Data deposited in or computed by PubChem | |
Molecular Formula |
C9H15O6P | |
Source | PubChem | |
URL | https://pubchem.ncbi.nlm.nih.gov | |
Description | Data deposited in or computed by PubChem | |
DSSTOX Substance ID |
DTXSID90208290 | |
Record name | Tris(2-carboxyethyl)phosphine | |
Source | EPA DSSTox | |
URL | https://comptox.epa.gov/dashboard/DTXSID90208290 | |
Description | DSSTox provides a high quality public chemistry resource for supporting improved predictive toxicology. | |
Molecular Weight |
250.19 g/mol | |
Source | PubChem | |
URL | https://pubchem.ncbi.nlm.nih.gov | |
Description | Data deposited in or computed by PubChem | |
CAS No. |
5961-85-3 | |
Record name | Tris(2-carboxyethyl)phosphine | |
Source | CAS Common Chemistry | |
URL | https://commonchemistry.cas.org/detail?cas_rn=5961-85-3 | |
Description | CAS Common Chemistry is an open community resource for accessing chemical information. Nearly 500,000 chemical substances from CAS REGISTRY cover areas of community interest, including common and frequently regulated chemicals, and those relevant to high school and undergraduate chemistry classes. This chemical information, curated by our expert scientists, is provided in alignment with our mission as a division of the American Chemical Society. | |
Explanation | The data from CAS Common Chemistry is provided under a CC-BY-NC 4.0 license, unless otherwise stated. | |
Record name | Tris(2-carboxyethyl)phosphine | |
Source | ChemIDplus | |
URL | https://pubchem.ncbi.nlm.nih.gov/substance/?source=chemidplus&sourceid=0005961853 | |
Description | ChemIDplus is a free, web search system that provides access to the structure and nomenclature authority files used for the identification of chemical substances cited in National Library of Medicine (NLM) databases, including the TOXNET system. | |
Record name | Tris(2-carboxyethyl)phosphine | |
Source | EPA DSSTox | |
URL | https://comptox.epa.gov/dashboard/DTXSID90208290 | |
Description | DSSTox provides a high quality public chemistry resource for supporting improved predictive toxicology. | |
Record name | TRIS(2-CARBOXYETHYL)PHOSPHINE | |
Source | FDA Global Substance Registration System (GSRS) | |
URL | https://gsrs.ncats.nih.gov/ginas/app/beta/substances/22OAC2MO2S | |
Description | The FDA Global Substance Registration System (GSRS) enables the efficient and accurate exchange of information on what substances are in regulated products. Instead of relying on names, which vary across regulatory domains, countries, and regions, the GSRS knowledge base makes it possible for substances to be defined by standardized, scientific descriptions. | |
Explanation | Unless otherwise noted, the contents of the FDA website (www.fda.gov), both text and graphics, are not copyrighted. They are in the public domain and may be republished, reprinted and otherwise used freely by anyone without the need to obtain permission from FDA. Credit to the U.S. Food and Drug Administration as the source is appreciated but not required. | |
Synthesis routes and methods
Procedure details
Retrosynthesis Analysis
AI-Powered Synthesis Planning: Our tool employs the Template_relevance Pistachio, Template_relevance Bkms_metabolic, Template_relevance Pistachio_ringbreaker, Template_relevance Reaxys, Template_relevance Reaxys_biocatalysis model, leveraging a vast database of chemical reactions to predict feasible synthetic routes.
One-Step Synthesis Focus: Specifically designed for one-step synthesis, it provides concise and direct routes for your target compounds, streamlining the synthesis process.
Accurate Predictions: Utilizing the extensive PISTACHIO, BKMS_METABOLIC, PISTACHIO_RINGBREAKER, REAXYS, REAXYS_BIOCATALYSIS database, our tool offers high-accuracy predictions, reflecting the latest in chemical research and data.
Strategy Settings
Precursor scoring | Relevance Heuristic |
---|---|
Min. plausibility | 0.01 |
Model | Template_relevance |
Template Set | Pistachio/Bkms_metabolic/Pistachio_ringbreaker/Reaxys/Reaxys_biocatalysis |
Top-N result to add to graph | 6 |
Feasible Synthetic Routes
Haftungsausschluss und Informationen zu In-Vitro-Forschungsprodukten
Bitte beachten Sie, dass alle Artikel und Produktinformationen, die auf BenchChem präsentiert werden, ausschließlich zu Informationszwecken bestimmt sind. Die auf BenchChem zum Kauf angebotenen Produkte sind speziell für In-vitro-Studien konzipiert, die außerhalb lebender Organismen durchgeführt werden. In-vitro-Studien, abgeleitet von dem lateinischen Begriff "in Glas", beinhalten Experimente, die in kontrollierten Laborumgebungen unter Verwendung von Zellen oder Geweben durchgeführt werden. Es ist wichtig zu beachten, dass diese Produkte nicht als Arzneimittel oder Medikamente eingestuft sind und keine Zulassung der FDA für die Vorbeugung, Behandlung oder Heilung von medizinischen Zuständen, Beschwerden oder Krankheiten erhalten haben. Wir müssen betonen, dass jede Form der körperlichen Einführung dieser Produkte in Menschen oder Tiere gesetzlich strikt untersagt ist. Es ist unerlässlich, sich an diese Richtlinien zu halten, um die Einhaltung rechtlicher und ethischer Standards in Forschung und Experiment zu gewährleisten.