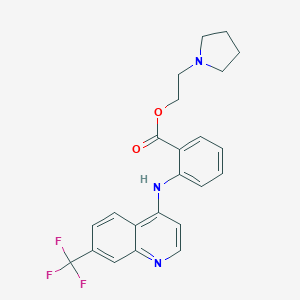
Florifenine
Übersicht
Beschreibung
Vorbereitungsmethoden
Synthesewege und Reaktionsbedingungen: Florifenin wird in einem mehrstufigen Verfahren synthetisiert, bei dem 7-(Trifluormethyl)-4-Chinolinamin mit 2-(1-Pyrrolidinyl)ethyl-Anthranilat umgesetzt wird . Die Reaktion erfordert typischerweise ein Lösungsmittel wie Dimethylformamid (DMF) und einen Katalysator wie Triethylamin. Die Reaktion wird unter Rückflussbedingungen durchgeführt, um eine vollständige Umwandlung der Reaktanten in das gewünschte Produkt zu gewährleisten .
Industrielle Produktionsmethoden: In industriellen Umgebungen beinhaltet die Produktion von Florifenin die großtechnische Synthese unter ähnlichen Reaktionsbedingungen, jedoch mit optimierten Parametern, um Ausbeute und Reinheit zu verbessern. Der Prozess umfasst strenge Reinigungsschritte wie Umkristallisation und Chromatographie, um hochreines Florifenin zu erhalten, das für pharmazeutische Anwendungen geeignet ist .
Analyse Chemischer Reaktionen
Reaktionstypen: Florifenin unterliegt verschiedenen chemischen Reaktionen, darunter:
Häufige Reagenzien und Bedingungen:
Hauptprodukte:
Wissenschaftliche Forschungsanwendungen
Florifenin hat eine breite Palette von Anwendungen in der wissenschaftlichen Forschung, darunter:
Biologie: Untersucht auf seine Auswirkungen auf zelluläre Prozesse wie Entzündungen und Zellmigration.
Industrie: Eingesetzt bei der Entwicklung von entzündungshemmenden Formulierungen und topischen Behandlungen.
5. Wirkmechanismus
Florifenin entfaltet seine entzündungshemmenden Wirkungen durch Hemmung der Aktivität von Cyclooxygenasen (COX), insbesondere COX-2 . Diese Hemmung führt zu einer Verringerung der Produktion von pro-inflammatorischen Mediatoren wie Prostaglandinen und Thromboxanen . Zusätzlich hemmt Florifenin die Neutrophilenmigration und die Elastasefreisetzung, was zu seinen entzündungshemmenden Eigenschaften beiträgt .
Wirkmechanismus
Florifenine exerts its anti-inflammatory effects by inhibiting the activity of cyclooxygenases (COX), particularly COX-2 . This inhibition leads to a decrease in the production of pro-inflammatory mediators such as prostaglandins and thromboxanes . Additionally, this compound inhibits neutrophil migration and elastase release, further contributing to its anti-inflammatory properties .
Vergleich Mit ähnlichen Verbindungen
Florifenin ist einzigartig in seiner Struktur durch das Vorhandensein einer Trifluormethylgruppe am Chinolinring, die seine entzündungshemmende Aktivität signifikant verstärkt . Ähnliche Verbindungen umfassen:
Naproxen: Ein weiteres NSAR mit einem ähnlichen Wirkmechanismus, aber einer anderen chemischen Struktur.
Celecoxib: Ein selektiver COX-2-Hemmer mit einem anderen strukturellen Aufbau.
Die einzigartigen Strukturmerkmale und die starke entzündungshemmende Wirkung von Florifenin machen es zu einer wertvollen Verbindung sowohl in der Forschung als auch in therapeutischen Anwendungen .
Biologische Aktivität
Florifenine, a fluorinated compound, has garnered interest in recent years for its potential biological activities, particularly in the fields of pharmacology and medicinal chemistry. This article explores the biological activity of this compound, focusing on its mechanisms of action, therapeutic applications, and relevant case studies.
Chemical Structure and Properties
This compound is characterized by its unique fluorinated structure, which influences its biological activity. The presence of fluorine atoms can enhance lipophilicity and metabolic stability, potentially improving the pharmacokinetic properties of the compound. Studies have shown that fluorination can significantly alter the conformational preferences of compounds, impacting their interaction with biological targets .
This compound's biological activity is primarily attributed to its ability to modulate various biochemical pathways. Research indicates that fluorinated compounds often exhibit distinct mechanisms of action compared to their non-fluorinated counterparts. For instance, this compound may interact with specific enzymes or receptors, leading to altered signaling pathways that affect cellular function.
Key Mechanisms:
- Inhibition of Enzymatic Activity : Some studies suggest that this compound may inhibit enzymes involved in inflammatory processes, similar to other fluorinated compounds that target phospholipase A2 .
- Antiproliferative Effects : this compound has demonstrated antiproliferative activity against various cancer cell lines, indicating potential as an anticancer agent .
Biological Activity and Therapeutic Applications
This compound's biological activity has been evaluated in several contexts, including anti-inflammatory and anticancer applications.
Anti-inflammatory Activity
In preclinical models, this compound has shown promise in reducing inflammation. For example, it has been tested in models of adjuvant-induced arthritis, where it demonstrated significant reductions in inflammatory markers .
Anticancer Activity
This compound's antiproliferative effects have been assessed against multiple cancer cell lines:
- Breast Cancer : In vitro studies revealed that this compound effectively inhibited the growth of breast cancer cells.
- Colon and Lung Cancer : Similar inhibitory effects were noted against colon and lung cancer cell lines, suggesting a broad spectrum of activity .
Case Studies
Several case studies highlight the therapeutic potential of this compound:
-
Case Study 1: Anti-inflammatory Effects
- In a study involving rats with induced arthritis, administration of this compound resulted in a dose-dependent decrease in paw swelling and inflammatory cytokines.
- Findings : The compound significantly reduced levels of TNFα and IL-6 in serum samples.
-
Case Study 2: Anticancer Efficacy
- A series of experiments evaluated the efficacy of this compound against different cancer cell lines.
- Results : The compound exhibited IC50 values in the micromolar range for breast and colon cancer cells, suggesting effective cytotoxicity.
Data Summary
The following table summarizes key findings related to the biological activity of this compound:
Eigenschaften
IUPAC Name |
2-pyrrolidin-1-ylethyl 2-[[7-(trifluoromethyl)quinolin-4-yl]amino]benzoate | |
---|---|---|
Source | PubChem | |
URL | https://pubchem.ncbi.nlm.nih.gov | |
Description | Data deposited in or computed by PubChem | |
InChI |
InChI=1S/C23H22F3N3O2/c24-23(25,26)16-7-8-17-20(9-10-27-21(17)15-16)28-19-6-2-1-5-18(19)22(30)31-14-13-29-11-3-4-12-29/h1-2,5-10,15H,3-4,11-14H2,(H,27,28) | |
Source | PubChem | |
URL | https://pubchem.ncbi.nlm.nih.gov | |
Description | Data deposited in or computed by PubChem | |
InChI Key |
DQOYUDHAZMAVLD-UHFFFAOYSA-N | |
Source | PubChem | |
URL | https://pubchem.ncbi.nlm.nih.gov | |
Description | Data deposited in or computed by PubChem | |
Canonical SMILES |
C1CCN(C1)CCOC(=O)C2=CC=CC=C2NC3=C4C=CC(=CC4=NC=C3)C(F)(F)F | |
Source | PubChem | |
URL | https://pubchem.ncbi.nlm.nih.gov | |
Description | Data deposited in or computed by PubChem | |
Molecular Formula |
C23H22F3N3O2 | |
Source | PubChem | |
URL | https://pubchem.ncbi.nlm.nih.gov | |
Description | Data deposited in or computed by PubChem | |
DSSTOX Substance ID |
DTXSID00868763 | |
Record name | 2-(Pyrrolidin-1-yl)ethyl 2-{[7-(trifluoromethyl)quinolin-4-yl]amino}benzoate | |
Source | EPA DSSTox | |
URL | https://comptox.epa.gov/dashboard/DTXSID00868763 | |
Description | DSSTox provides a high quality public chemistry resource for supporting improved predictive toxicology. | |
Molecular Weight |
429.4 g/mol | |
Source | PubChem | |
URL | https://pubchem.ncbi.nlm.nih.gov | |
Description | Data deposited in or computed by PubChem | |
CAS No. |
83863-79-0 | |
Record name | Florifenine [INN] | |
Source | ChemIDplus | |
URL | https://pubchem.ncbi.nlm.nih.gov/substance/?source=chemidplus&sourceid=0083863790 | |
Description | ChemIDplus is a free, web search system that provides access to the structure and nomenclature authority files used for the identification of chemical substances cited in National Library of Medicine (NLM) databases, including the TOXNET system. | |
Record name | 2-(Pyrrolidin-1-yl)ethyl 2-{[7-(trifluoromethyl)quinolin-4-yl]amino}benzoate | |
Source | EPA DSSTox | |
URL | https://comptox.epa.gov/dashboard/DTXSID00868763 | |
Description | DSSTox provides a high quality public chemistry resource for supporting improved predictive toxicology. | |
Record name | FLORIFENINE | |
Source | FDA Global Substance Registration System (GSRS) | |
URL | https://gsrs.ncats.nih.gov/ginas/app/beta/substances/S7UT8VQG94 | |
Description | The FDA Global Substance Registration System (GSRS) enables the efficient and accurate exchange of information on what substances are in regulated products. Instead of relying on names, which vary across regulatory domains, countries, and regions, the GSRS knowledge base makes it possible for substances to be defined by standardized, scientific descriptions. | |
Explanation | Unless otherwise noted, the contents of the FDA website (www.fda.gov), both text and graphics, are not copyrighted. They are in the public domain and may be republished, reprinted and otherwise used freely by anyone without the need to obtain permission from FDA. Credit to the U.S. Food and Drug Administration as the source is appreciated but not required. | |
Retrosynthesis Analysis
AI-Powered Synthesis Planning: Our tool employs the Template_relevance Pistachio, Template_relevance Bkms_metabolic, Template_relevance Pistachio_ringbreaker, Template_relevance Reaxys, Template_relevance Reaxys_biocatalysis model, leveraging a vast database of chemical reactions to predict feasible synthetic routes.
One-Step Synthesis Focus: Specifically designed for one-step synthesis, it provides concise and direct routes for your target compounds, streamlining the synthesis process.
Accurate Predictions: Utilizing the extensive PISTACHIO, BKMS_METABOLIC, PISTACHIO_RINGBREAKER, REAXYS, REAXYS_BIOCATALYSIS database, our tool offers high-accuracy predictions, reflecting the latest in chemical research and data.
Strategy Settings
Precursor scoring | Relevance Heuristic |
---|---|
Min. plausibility | 0.01 |
Model | Template_relevance |
Template Set | Pistachio/Bkms_metabolic/Pistachio_ringbreaker/Reaxys/Reaxys_biocatalysis |
Top-N result to add to graph | 6 |
Feasible Synthetic Routes
Q1: What is the primary area of focus regarding Florifenine's activity according to this research paper?
A1: This study primarily investigates this compound's potential as a topical anti-inflammatory agent. The research explores its effects on arachidonic acid metabolism and neutrophil functions, both of which are key players in inflammatory processes [].
Q2: Can you elaborate on the significance of studying arachidonic acid metabolism and neutrophil functions in relation to this compound's potential as an anti-inflammatory agent?
A2: Arachidonic acid is a fatty acid that serves as a precursor to various inflammatory mediators, such as prostaglandins and leukotrienes. Neutrophils are immune cells that play a critical role in the early stages of inflammation. By investigating this compound's influence on arachidonic acid metabolism and neutrophil functions, the researchers aim to understand the compound's mechanisms of action in mitigating inflammation [].
Haftungsausschluss und Informationen zu In-Vitro-Forschungsprodukten
Bitte beachten Sie, dass alle Artikel und Produktinformationen, die auf BenchChem präsentiert werden, ausschließlich zu Informationszwecken bestimmt sind. Die auf BenchChem zum Kauf angebotenen Produkte sind speziell für In-vitro-Studien konzipiert, die außerhalb lebender Organismen durchgeführt werden. In-vitro-Studien, abgeleitet von dem lateinischen Begriff "in Glas", beinhalten Experimente, die in kontrollierten Laborumgebungen unter Verwendung von Zellen oder Geweben durchgeführt werden. Es ist wichtig zu beachten, dass diese Produkte nicht als Arzneimittel oder Medikamente eingestuft sind und keine Zulassung der FDA für die Vorbeugung, Behandlung oder Heilung von medizinischen Zuständen, Beschwerden oder Krankheiten erhalten haben. Wir müssen betonen, dass jede Form der körperlichen Einführung dieser Produkte in Menschen oder Tiere gesetzlich strikt untersagt ist. Es ist unerlässlich, sich an diese Richtlinien zu halten, um die Einhaltung rechtlicher und ethischer Standards in Forschung und Experiment zu gewährleisten.